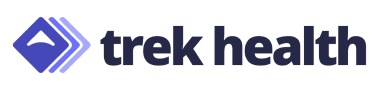
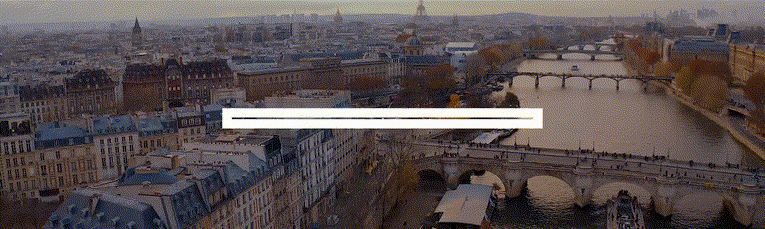
Maximize Practice Revenue with Smart Payment Solutions
Enhance your financial performance with our cutting-edge RCM and Payer Transparency solutions—designed for precision, automation, and minimal administrative overhead. Start realizing increased revenue with smarter, more efficient billing processes.
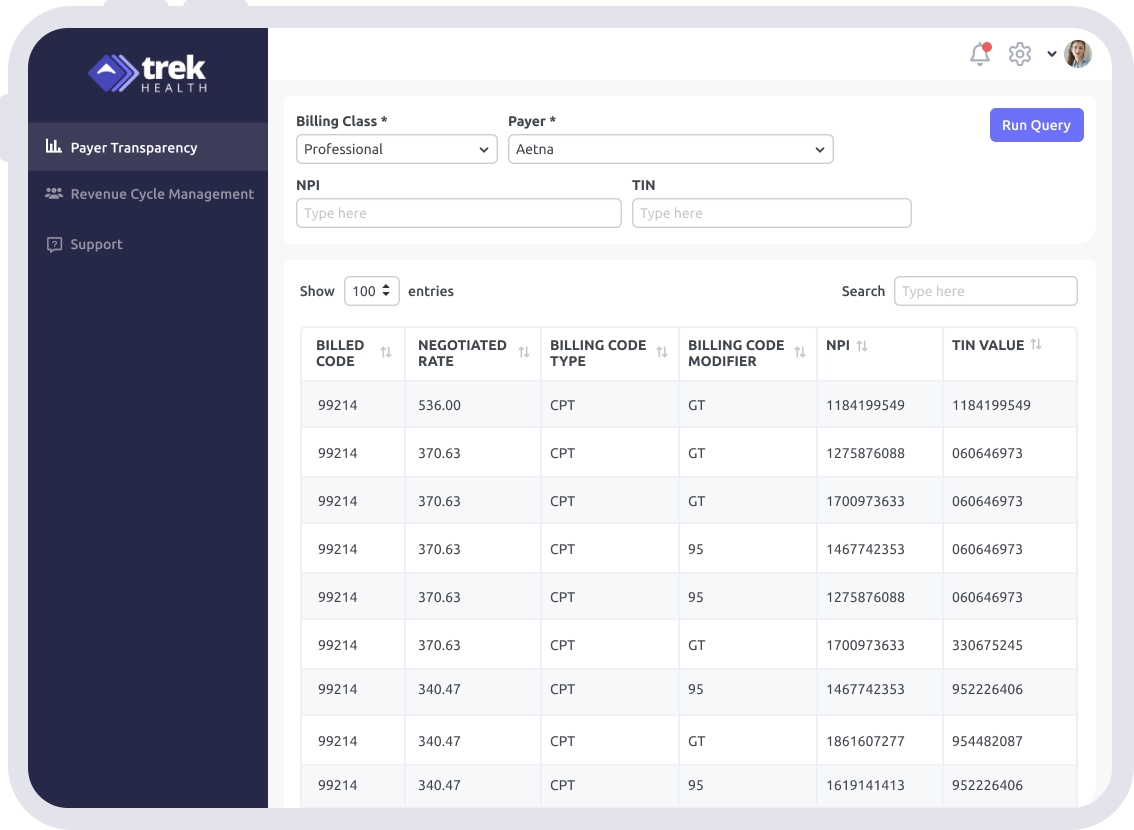
Optimize Healthcare Financials, Enhance Patient Care
Unify financial oversight and patient engagement with Trek Health. Our dual solutions in RCM and Payer Transparency streamline payment cycles and unlock market insights, enhancing revenue while you provide exceptional care.
Insightful Data for Strategic Decisions
Harness Market Clarity
Navigate payer landscapes with confidence. Our Payer Transparency tool delivers real-time analytics and market insights, empowering you to make data-driven decisions and optimize financial outcomes.
Comprehensive Market Analysis
Real-Time Rate Comparisons
Enhance Payer Negotiations
.webp)
Your Revenue, Streamlined
Optimize Your Financial Health
Elevate your financial performance with our RCM system. Experience a seamless flow from patient entry to final payment, backed by automation that reduces errors and enhances revenue collection.
Automated Billing and Collections
Denial Management Intelligence
Patient Payment Simplification
Payer Reported Contracted Rates
Average Insurance Reimbursement Rate Across Customers
Providers Served Across Customers
From Insight to Impact: A Streamlined Financial Journey
Connect the dots from market analysis to optimized revenue cycles.
Market Insight & Strategy Development
Enhancing Negotiation & Credentialing Workflows
Operational Workflow Design
RCM Implementation
Financial Performance &Growth Monitoring
Our integrated solutions ensure a cohesive financial management strategy that enhances every step from payer negotiations to patient payments, fostering a healthy financial ecosystem for your practice.
Ready to See Trek Health in Action?
A series of blogs on inspiration and events of trek health.
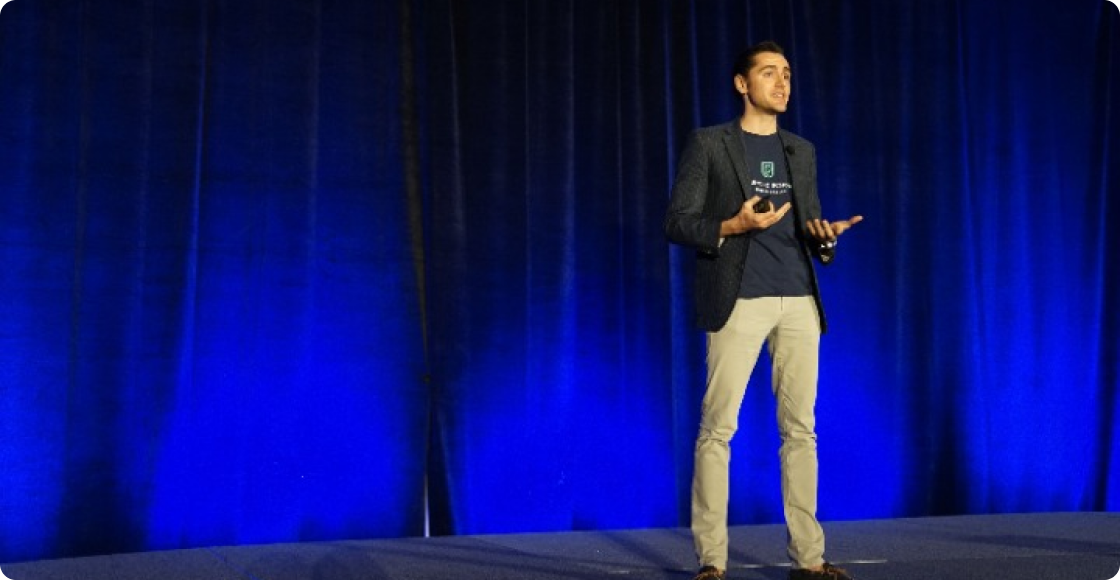
How to Bill Clients with Confidence as a Mental Health Practitioner
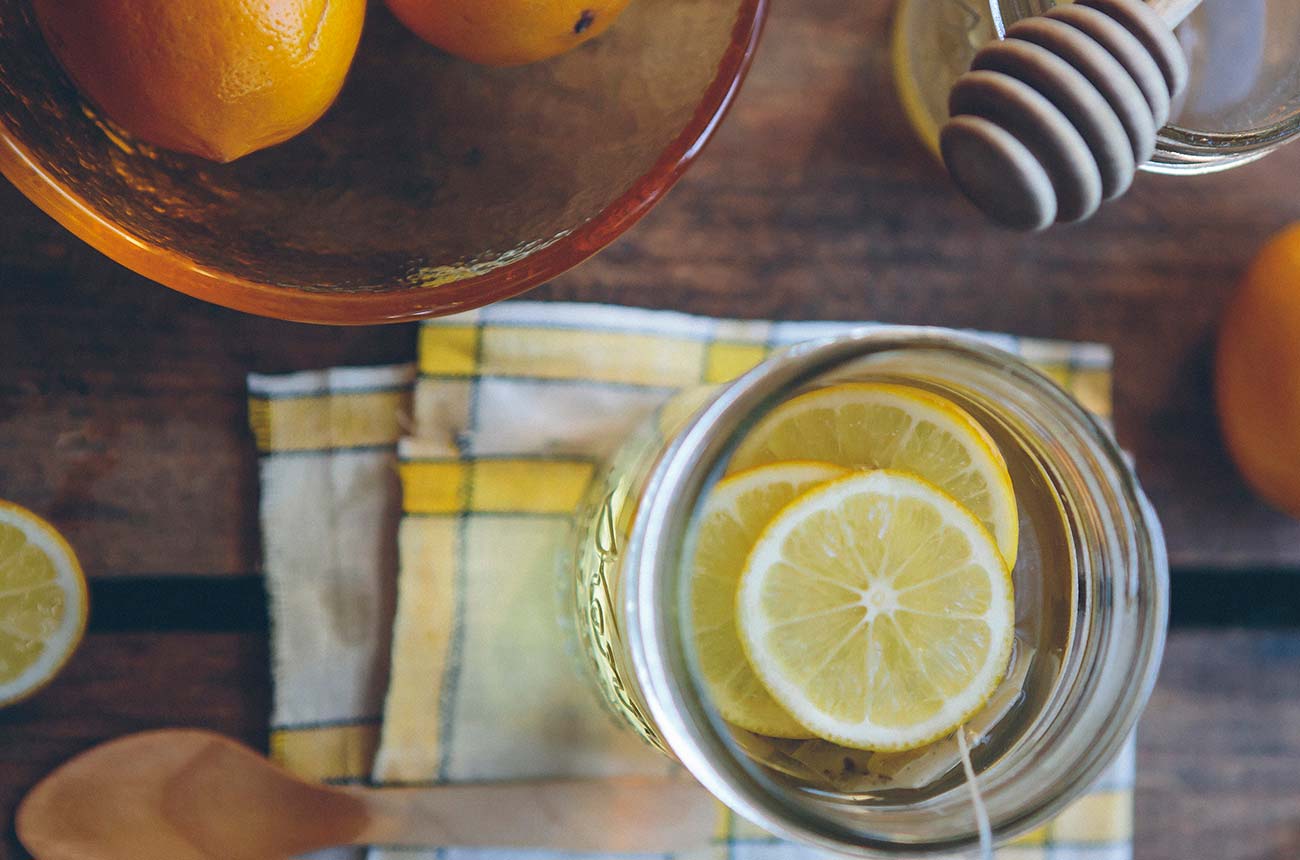
Provident Voluptates Ducimus
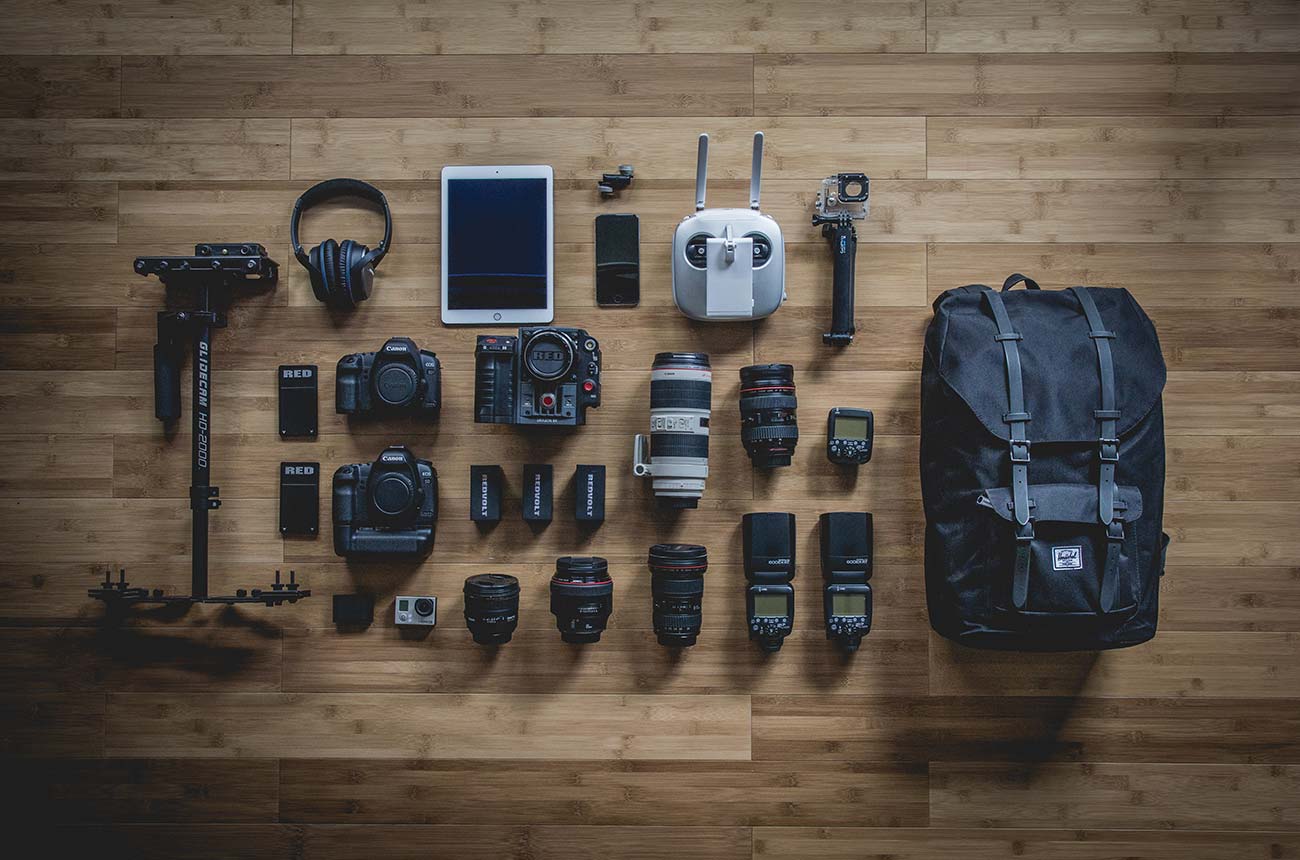
Laudantium Non Ad

An official website of the United States government
The .gov means it’s official. Federal government websites often end in .gov or .mil. Before sharing sensitive information, make sure you’re on a federal government site.
The site is secure. The https:// ensures that you are connecting to the official website and that any information you provide is encrypted and transmitted securely.
- Publications
- Account settings
Preview improvements coming to the PMC website in October 2024. Learn More or Try it out now .
- Advanced Search
- Journal List
- Front Pharmacol
Role of TREK-1 in Health and Disease, Focus on the Central Nervous System
TREK-1 is the most studied background K 2P channel. Its main role is to control cell excitability and maintain the membrane potential below the threshold of depolarization. TREK-1 is multi-regulated by a variety of physical and chemical stimuli which makes it a very promising and challenging target in the treatment of several pathologies. It is mainly expressed in the brain but also in heart, smooth muscle cells, endocrine pancreas, and prostate. In the nervous system, TREK-1 is involved in many physiological and pathological processes such as depression, neuroprotection, pain, and anesthesia. These properties explain why many laboratories and pharmaceutical companies have been focusing their research on screening and developing highly efficient modulators of TREK-1 channels. In this review, we summarize the different roles of TREK-1 that have been investigated so far in attempt to characterize pharmacological tools and new molecules to modulate cellular functions controlled by TREK-1.
Introduction
In the brain, ion channels regulate a variety of cellular processes such as neurotransmitter release, neuronal excitability, and plasticity. They are crucial in the generation and propagation of action potentials. Dysfunction of these channels causes several pathologies called channelopathies. In order to treat these channelopathies, many ion channels have been targets of small molecules and biological drugs. Overall, ion channels represent 19% of all human protein targets ( Santos et al., 2017 ). To date, 177 ion channels-based drugs have been approved as treatments for various pathologies and disorders ( Santos et al., 2017 ). Central nervous system (CNS) pathologies were associated with dysregulation of ion channels such as voltage gated Na + , K + , and Ca 2+ channels. Calcium channels are the most studied among ion channels since Ca 2+ ions in addition to controlling the cell excitability, they act as second messengers that convert electrical signals into chemical activity ( Pietrobon, 2002 ). On the other hand, to control neuronal excitability, potassium channels repolarize neurons by extruding K + ions to the extracellular space. Neurological potassium channelopathies are mainly caused by dysregulation of voltage-dependent potassium channels ( Benatar, 2000 ) and also of the inwardly rectifying potassium channels (Kir) which provokes an hyperexcitability seen in epilepsy ( Spillane et al., 2016 ). Mutations in the KCNT1 gene encoding for sodium -activated potassium channels cause a rare infantile encephalopathy called the migrating partial seizures of infancy ( Barcia et al., 2012 ). In other cases, mutations of KCNQ2 encoding for Kv7.2 channel provoke severe epileptic encephalopathies ( Weckhuysen et al., 2012 ). The inward-rectifier potassium channel Kir 6.2 is encoded by KCNJ11 gene forms the major subunit of the ATP-sensitive potassium channel. Mutations in this gene cause DEND syndrome, a very rare severe form of neonatal diabetes mellitus characterized by developmental delay, epilepsy and neonatal diabetes ( Gloyn et al., 2004 ). Kv7.2 and Kv7.3 are the main components of the slow voltage-gated M-channel which regulates neuronal excitability ( Brown and Passmore, 2009 ). Loss of function mutations of the KCNQ2 and KCNQ3 genes encoding, respectively, for Kv7.2 and Kv7.3 causes benign familial neonatal convulsions ( Spillane et al., 2016 ). A dominant missense mutation in KCNMA1 gene encoding for pore-forming α-subunit of the large conductance calcium-sensitive potassium channel (BK), has been associated with a form of generalized epilepsy and paroxysmal dyskinesia ( Du et al., 2005 ).
Some mutations in K 2P channels were reported to cause several pathologies. Birk Barel mental retardation dysmorphism syndrome is caused by a missense mutation in the maternal copy of KCNK9 gene which encodes for TASK-3 (K 2P 9.1). Loss of function of the channel was observed in homodimers and also when it forms heterodimer with TASK-1 (K 2P .3.1) ( Barel et al., 2008 ). Recently, de novo a gain of function missense mutation of KCNK4 gene encoding for TRAAK channel, was reported to cause recognizable neurodevelopmental syndrome characterized by a facial dysmorphism, hypertrichosis, epilepsy, intellectual disability/developmental delay and gingival overgrowth ( Bauer et al., 2018 ). A frameshift mutation (F139Wfsx24) in KCNK18 encoding for the calcium-activated K 2P channel TRESK channel was associated with migraine with aura ( Lafreniere et al., 2010 ). Channelopathies caused by dysfunction of potassium channels are of high interest for researchers since they may present interesting targets for potential treatments.
The most recent discovered family of two-pore domain potassium channels (K 2P ) are regulated by a variety of chemical and physical stimuli ( Lesage and Lazdunski, 2000 ). In this review, we will focus on describing the role of one of the most studied K 2P channel, TREK-1 channel in health and disease. In addition to discussing the recent pharmacological modulators of its activity.
Trek-1 Channel
TREK-1, named KCNK2 or K 2P 2.1 belongs to a large family of K 2P channels containing 15 members grouped in six subfamilies. K 2P channels are the most recent class of K + channels discovered. K 2P channels or the two-pore domain potassium channels are tandems of four transmembrane segments (M1–M4) containing two-pore domain (P1 and P2) ( Figure 1 , ,2). 2 ). They possess an extended M1-P1 extracellular loop and cytosolic N- and C-termini. K 2P channels have a unique pore signature sequence Gly-Tyr(Phe)-Gly in the 1st pore (P1) and Gly-Leu(Phe)-Gly in the 2nd pore (P2) ( Honore, 2007 ; Figure 1 ). TREK-1 was first cloned from the mouse brain ( Fink et al., 1996 ; Figure 3 ). TREK-1 was named after TWIK-1 channel the first cloned K 2P channel ( Lesage et al., 1996 ). TREK-1 shares 28% sequence homology with TWIK-1 channel. TREK-1 is highly expressed in brain and lung, but is also present in kidney, heart and skeletal muscle. When we look at the brain localization, TREK-1 is highly expressed in several regions of the brain such as the olfactory bulb, the hippocampus, the cerebellum and the cortex ( Fink et al., 1996 ).

Structure and classification of K 2P channels. The family of K 2P channels is composed of 15 members grouped in six subfamilies. K 2P channels are two-pore domain potassium channels and the most recent class of K + channels discovered. They assemble as dimers of four transmembrane segments (M1–M4) and two-pore domain (P1 and P2). They have an extended M1-P1 extracellular loop and cytosolic N- and C-termini. K 2P channels have a unique pore signature sequence Gly-Tyr(Phe)-Gly in the 1st pore (P1) and Gly-Leu(Phe)-Gly in the 2nd pore (P2).

Polymodal TREK-1 regulation. TREK-1 is multiregulated by a variety of physical and chemical stimuli. TREK-1 possesses different protein partners such as AKAP150, β-COP, Mtap2, and sortilin. Sortilin interacts with TREK-1 an address it to the plasma membrane. Spadin is a synthetic peptide derived from sortilin which was shown to block TREK-1 with high affinity. Spadin antidepressant activity appears to be mediated through PI3K and Akt activation. TREK-1 is involved in numerous CNS pathologies such as depression, ischemia, epilepsy and pain.

Key milestones in discovery of TREK-1 channels. The scheme presents the major dates from the cloning of TREK-1 channels to the discovery of its role in physiology and pathology. AA, arachidonic acid; GPCR, G-protein Coupled Receptor; STAR ∗ D, Sequenced Treatment Alternatives to Relieve Depression. Green and Red triangles represent TREK-1 activation and inhibition processes, respectively.
TREK-1 displays an outward rectification in symmetrical K + condition due to an external Mg 2+ block present at negative potential and to a voltage-dependence mechanism ( Maingret et al., 2002 ). Despite the absence of a voltage-sensing domain in K 2P channels, TREK-1 and some other K 2P channels show a strong voltage-dependency. The provenance of this voltage sensitivity comes from an ion-flux gating mechanism and the movement of three to four K + ions into the high electric field of an inactive selectivity filter ( Schewe et al., 2016 ). However, this voltage-dependency is switched off by physiological stimuli such as arachidonic acid (AA) and Phosphatidylinositol bisphosphate (PIP 2 ), which convert TREK-1 to classical leak channels ( Schewe et al., 2016 ). TREK-1 with TREK-2 and TRAAK are mechano- and thermo-sensitive K 2P channels ( Honore, 2007 ). They are opened by stretch and cell swelling. TREK-1, TREK-2, and TRAAK knock-out mice are hypersensitive to mechanical force, they show mechanical allodynia and hyperalgesia during inflammation ( Brohawn, 2015 ). It was reported that the mechanical force is transmitted to TREK-1 and TRAAK directly through the lipid bilayer ( Brohawn et al., 2014 ). These channels open rapidly in response to tension and have a low threshold and broad range of tension activation ( Brohawn, 2015 ).
Physiological Regulation of TREK-1 Channels
TREK-1 channel is regulated by various physical and chemical stimuli ( Figure 2 ). TREK-1 is opened by mechanical stretch in cell-attached and inside-out configurations in both COS transfected cells and oocytes ( Patel et al., 1998 ). TREK-1 opening occurs at all positive and negative potentials. When the cytoskeleton is disrupted with colchicine or cytochalasin D, TREK-1 activation by stretch was not altered ( Patel et al., 1998 ). TREK-1 was shown to be sensitive to temperature variation in different type of cells expressing TREK-1. Heat gradually and reversibly activates TREK-1 reaching a maximum of activation at 37°C. However, at 12°C TREK-1 basal current is suppressed ( Maingret et al., 2000 ). TREK-1 heat activation needs cell integrity and cytosolic components since TREK-1 is insensitive to heat when patch membrane is excised ( Maingret et al., 2000 ). TREK-1 is activated by polyunsaturated fatty acids (PUFA) such as AA in different patch-clamp configurations: whole-cell, cell-attached, inside-out and outside-out configurations ( Patel et al., 1998 ). AA activates TREK-1 with a dose-dependent manner and requires C-terminal part of the channel which is crucial for the activation and the inhibition following phosphorylation by the protein kinase A (PKA). PIP 2 stimulates native striatal TREK-1 current in inside-out patch configuration ( Chemin et al., 2005 ). In Xenopus oocytes expressing TREK-1, PIP 2 hydrolysis inhibits TREK-1 channel by modulating its voltage dependence ( Lopes et al., 2005 ). It is also known that internal acidosis induces an activation of TREK-1 channels with the involvement of C-terminal part ( Maingret et al., 1999 ). TREK subfamily members including TREK-1, TREK-2, and TRAAK are sensitive to phosphatidic acid (PA) which results from the hydrolysis of phosphatidylcholine by phospholipase D (PLD). It was shown that TREK-1 and TREK-2 but not TRAAK are positively regulated by PLD2 which selectively binds to their C-terminal domains ( Comoglio et al., 2014 ).
TREK-1 Partner Proteins
Proteomic approach based on immunoprecipitation and mass spectrometry analysis of native channel complexes allowed the identification of a scaffolding protein called AKAP150 ( Sandoz et al., 2006 ; Figure 2 ). A-kinase anchoring protein (AKAP) anchors the regulatory subunit of PKA in proximity of substrates. AKAP150 forms signaling complexes within the neuron, these complexes are composed of PKA, PKC, PP2B (protein phosphatase 2B), PSD-95, SAP97 and ion channels ( Esseltine and Scott, 2013 ). When anchored by AKAP150 within the post-M4 region, TREK-1 becomes an active leak channel insensitive to the stimuli, internal acidification AA, or mechanical stretch ( Sandoz et al., 2006 ). The microtubule associated protein Mtap2 is another protein reported to associate with TREK-1 in the brain ( Sandoz et al., 2008 ). Mtap2 binds to microtubules and stabilizes them. TREK-1 and Mtap2 colocalize in several brain regions such as the hippocampus, cerebellum, olfactory bulb, striatum and cortex. Similarly to AKAP150, Mtap2 binding to TREK-1 increases its activity ( Sandoz et al., 2008 ). This increase in TREK-1 current amplitude is not due to a direct interaction with Mtap2 but results from an increase in the expression level of the channel at the plasma membrane. Within the neuron, TREK-1, AKAP150 and Mtap2 are simultaneously found in the post-synaptic terminals. This signaling complex regulates TREK-1 channel activity and its trafficking to the plasma membrane.
Using the yeast two-hybrid technique of the human cDNA library on the N-terminal region of TREK-1, a direct β-COP-TREK-1 interaction was reported ( Kim et al., 2010 ). β-COP is a subunit of Coat Protein Complex I (COPI) whose role is to form coated vesicles and manage the retrograde traffic from the Golgi back to the endoplasmic reticulum (ER) or between different compartments inside the Golgi ( Gomez-Navarro and Miller, 2016 ). β-COP depletion was found to decrease membrane expression of the cystic fibrosis transmembrane conductance regulator (CFTR) channel. Mutations in this chloride channel cause cystic fibrosis lung disease ( Rennolds et al., 2008 ). β-COP increases TREK-1 surface expression and current amplitude. β-COP is involved in the forward transport of TREK-1 through a direct interaction with N-terminal part of the channel ( Kim et al., 2010 ).
The most recently discovered protein partner of TREK-1 is sortilin ( Petersen et al., 1997 ; Figure 2 ), also known as neurotensin receptor-3 (NTSR 3 ) ( Mazella et al., 1998 ). Sortilin is synthetized as a precursor called prosortilin which is cleaved in the trans-Golgi compartment by the proprotein convertase furin to generate a mature sortilin and the release of a 44 amino-acid named the propeptide (PE) ( Munck Petersen et al., 1999 ). NTSR 3 is made of a large luminal domain, a single transmembrane segment and a short C-terminal moiety. The amount of NTSR 3 expressed at the plasma membrane does not exceed 10% while the majority is expressed intracellularly and involved mainly in intracellular trafficking. Both NTSR 3 and TREK-1 are markedly expressed in the prefrontal (PFC) and cingulate cortex, the amygdala, the hippocampus, the nucleus accumbens, the dorsal raphe nucleus (DRN) and the hypothalamus. The expression of TREK-1 was enhanced at the plasma membrane in COS-7 cells co-transfected with NTSR 3 ( Mazella et al., 2010 ).
TREK-1 Heterodimerization
The first K 2P member cloned TWIK-1 ( Lesage et al., 1996 ), is expressed with TREK-1 in astrocytes ( Zhou et al., 2009 ). The expression of a functional TWIK-1 channel necessitates its heterodimerization with TREK-1 to mediate the passive conductance in astrocytes ( Hwang et al., 2014 ). As cited earlier in this review, TREK subfamily includes TREK-1, TREK-2 and TRAAK. Despite 78% of homology between TREK-1 and TREK-2, these two lipid- and mechano-activated K + channels are differently regulated. Recent studies reported that functional TREK-1 heterodimers are formed with TREK-2 and TRAAK channels ( Blin et al., 2016 ; Levitz et al., 2016 ). The heterodimer TREK-1-TREK-2 had minimal activity at physiological pH. However, TREK-1-TREK-2 complex is activated in both alkaline and acidic environments. TREK-1-TRAAK heterodimer shows activation by both intracellular acidification and alkalinization ( Levitz et al., 2016 ). Functional TREK-1-TRAAK heterodimers are formed and present unique biophysical properties and different type of regulations ( Blin et al., 2016 ). Heteromerization of TREK subfamily leads to diversification of K 2P channels and provides different kinds of regulations. Recently, it has been shown that a truncated form (TRESK-MT) of TRESK channel, a K 2P frequently associated with migraine, heteromerizes with and inhibits TREK-1 and TREK-2 ( Royal et al., 2019 ). The heterodimer TRESK-TREK acts as a dominant negative increasing trigeminal sensory neuron excitability and migraine-like phenotype in mice ( Royal et al., 2019 ).
Pharmacology of TREK-1 Channels
Trek-1 blockers.
Since the discovery of the involvement of TREK-1 in several CNS pathologies, very high interest has been devoted to find molecules modulators of its activity. As depicted in Table 1 , search for blockers of TREK-1 activity started with the discovery of the role of TREK-1 in depression processes. Mice model of depression lacking kcnk2 (the gene encoding for TREK-1) displays a phenotype resistant to the development of depression ( Heurteaux et al., 2006 ). Spadin was the first molecule that was developed in the aim to block TREK-1 channel and mimic the antidepressant-like phenotype of kcnk2 -/- mice ( Mazella et al., 2010 ; Djillani et al., 2018 ). Spadin is a peptide synthetized from the endogenous PE released after the cleavage of prosortilin by furin. Several analogs of spadin were developed afterward to improve spadin in vivo stability and antidepressant activity ( Veyssiere et al., 2015 ; Djillani et al., 2017 ). From a library of 22 peptides, the shorter peptide sequence PE 22–28 exhibited higher inhibition potency for TREK-1 associated with an improved stability in vivo and antidepressant properties ( Djillani et al., 2017 ). Similar properties were found in its biotinylated product or analog G/A-PE 22–28 (where Gly is replaced by an Ala). These spadin analogs show an extremely high affinity for TREK-1 as inhibition occurs at nanomolar concentrations. More interesting, spadin and analogs are specific blockers for TREK-1 as no effect was observed on both TREK-2 and TRAAK, the two closest K 2P members to TREK-1 which belong to the same subfamily and share almost 80% of homology ( Moha Ou Maati et al., 2012 ; Djillani et al., 2017 ).
TREK-1 blockers.
Selective Serotonin Reuptake Inhibitors (SSRI) is a family of small molecules with a high affinity for the serotonin transporter, SERT ( Hirano et al., 2005 ). SERT blockade generates an accumulation of serotonin in the synaptic clefts and displays antidepressant effects in clinic. In addition, at clinical concentrations, SSRI and fluoxetine antagonize TREK-1 channel (IC 50 = 19 μM for fluoxetine and IC 50 = 9 μM for Norfluoxetine, its active metabolite) ( Kennard et al., 2005 ). Other SSRIs such as paroxetine, citalopram or escitalopram also block TREK-1 with different potencies. However, SSRIs are not specific for TREK-1 since they target TREK-2, Nav1.5, and L-type Ca 2+ channels ( Wong et al., 2005 ; Poulin et al., 2014 ; Mcclenaghan et al., 2016 ).
Antipsychotic drugs used to treat psychosis, like schizophrenia and bipolar disorder, were shown to be potent blockers of TREK-1 channels. They antagonize TREK-1 and TREK-2 but do not affect TRAAK channels ( Table 1 ; Thummler et al., 2007 ). TREK-1 blockade was observed with both typical and atypical antipsychotic. For example, chlorpromazine and Loxapine block dose-dependently TREK-1 channels with IC 50 of 2.7 and 19.7 μM ( Thummler et al., 2007 ). These data demonstrate a potential link between TREK-1, schizophrenia and bipolar disorder.
Dihydropyridine analogs such as amlodipine or niguldipine are L-type Ca 2+ channel blockers. It has been shown that they also block TREK-1 with high affinity (IC 50 = 0.43 and 0.75 μM for amlodipine and niguldipine, respectively). In addition, mice lacking the L-type channel Cav1.3 display an antidepressant-like phenotype ( Busquet et al., 2010 ). The compound SID1900 blocks TREK-1 with an IC 50 ∼30 μM and was shown to produce antidepressant-like properties in a rat model of chronic unpredictable mild stress (CUMS) ( Ye et al., 2015 ). SID1900 effect was comparable to spadin. Still there is no evidence about other possible targets of SID1900 and more data are needed to conclude about its specificity. Finally, other molecules were reported to block TREK-1 channels and provide neuroprotection against ischemia and stroke such as 3-n-butylphtalide (NBP) and its analog lig4-4 ( Ji et al., 2011 ; Wang et al., 2018 ). However, if information is lacking about the specificity of NBP, lig4-4 was reported to affect hERG channel, voltage-gated K + channels (K v ), neuronal Na + and Ca 2+ channels, which could limit its development as potential neuroprotective molecule.
TREK-1 Activators
TREK-1 channel is activated by volatile general anesthetics such as chloroform, diethyl ether, halothane and isoflurane and it was shown that the C-terminal part is critical for its activation ( Table 2 ; Patel et al., 1999 ). If the chloroform seems to be selective for TREK-1 channels with an EC 50 (0.2–1.6 mM), halothane and isoflurane activate both TREK-1 and TASK channels. Furthermore, diethyl ether opens TREK-1 but decrease TASK channel activity ( Patel et al., 1999 ). In contrast to general anesthetics, local anesthetics like bupivacaine behave as inhibitors of TREK-1 ( Kindler et al., 1999 ). AA and other PUFAs open TREK-1 channels in dose-dependent manner ( Patel et al., 1998 ). Channel opening by AA, α-linolenic acid (ALA), and docosahexaenoic acid (DHA) is thought to contribute to neuroprotection ( Lauritzen et al., 2000 ). If SSRI antidepressants and antipsychotics drugs act as TREK-1 blockers, mood stabilizers such as lithium chloride and antiepileptics drugs like gabapentine, valproate and carbamazepine were reported to activate TREK-1 channel ( Kim et al., 2017 ). The tetrazole based compound BL-1249 showed an interesting TREK-1 activation with an EC 50 of around 1.5 μM in vitro in cultured human urinary bladder myocytes ( Tertyshnikova et al., 2005 ). In a pancreatic carcinoma cell line, BL-1249 activates dose-dependently TREK-1 with an IC 50 = 2 ± 2 μM ( Sauter et al., 2016 ). Recent studies provided more insights into the action mechanism of BL-1249. Indeed, it was shown that BL-1249 activates the selectivity filter C-type gate and activates selectively TREK-1 and TREK-2 channels 10-fold stronger than TRAAK channels ( Pope et al., 2018 ). In addition, BL-1249 action requires the C-terminal tail of the channel for the activation and the transmembrane domains M2 and M3 are critical for its selectivity ( Pope et al., 2018 ). Following the screening of more than 106,000 small molecules, an activator of TREK-1 named ML67 and its optimized analog ML67-33 were identified ( Bagriantsev et al., 2013 ). Then after, two molecules with higher potency were recently developed by the same laboratory, ML335 and ML402 which potentiate TREK-1 activity with EC 50 of 5.2 ± 0.8 μM and 5.9 ± 1.6 μM, respectively ( Table 2 ; Lolicato et al., 2017 ). ML335 and ML402 bind and activate a cryptic binding pocket within the C-type gate selectivity filter of TREK-1 channel ( Lolicato et al., 2017 ). Non-steroid anti-inflammatory drugs (NSAIDs) such as flufenamic acid, niflumic acid and mefenamic acid were reported to activate TREK-1 channels independently of cyclooxygenase COX inhibition ( Veale et al., 2014 ). Caffeic acid derivatives were reported to activates TREK-1 channel and provide potent analgesic effect in vivo ( Rodrigues et al., 2014 ; Vivier et al., 2017 ). Finally, small molecule GI-530159 was described as TREK-1 opener which reduces small DRG neurons excitability ( Loucif et al., 2018 ). However, GI-530159 is not selective for TREK-1 channels since it activates also TREK-2 with no significant effect on TRAAK channels ( Loucif et al., 2018 ).
TREK-1 activators.
TREK-1 Modulators
Riluzole ( Table 2 ) is a neuroprotective molecule marketed as an anticonvulsant drug. Its action mechanism involves the blockade of glutamate receptors. It is also prescribed to prolong the survival of patient suffering from amyotrophic lateral sclerosis. Besides, riluzole was shown to act as a transient TREK-1 activator within 30 s and strong inhibitor after 90 s ( Duprat et al., 2000 ). The dual activity on TREK-1 is thought to be mediated through cyclic Adenosine MonoPhosphate (cAMP) activation of PKA. On the other hand, riluzole produces a sustained activation of TRAAK without an inhibition.
TREK-1 and CNS Disorders
TREK-1 channels show a widespread distribution in rat and mouse brains ( Hervieu et al., 2001 ; Talley et al., 2001 ). It is rational to imagine multiple roles that TREK-1 can play in the CNS. In this part of the review, we will explain in depth why TREK-1 channel became a very promising target in numbers of pathologies that affect the CNS ( Figure 3 ).
TREK-1 in Depression
The role of TREK-1 in depression was demonstrated in mice invalidated for kcnk2 , the gene encoding for TREK-1 ( Heurteaux et al., 2006 ). TREK-1 knock-out mice displayed a phenotype resistant to depression in five different mouse models of depression: the Porsolt forced swim test (FST), the tail suspension test (TST), the conditioned suppression of motility test (CSMT), the learned helplessness test (LH), and the novelty-suppressed feeding test (NSF) ( Table 3 ; Heurteaux et al., 2006 ). The behavior of kcnk2 -/- mice was similar to those treated acutely or chronically with classical antidepressants like fluoxetine ( Heurteaux et al., 2006 ). Kcnk2 -deficient mice show an increase in serotonin (5-HT) neurotransmission in the DRN neurons. Deletion of TREK-1 increases neurogenesis induced with a chronic treatment with antidepressants ( Heurteaux et al., 2006 ). Blocking TREK-1 has become a novel strategy to design new generation of antidepressants. At the concentrations used clinically, SSRIs inhibits TREK-1 channels ( Kennard et al., 2005 ).
TREK-1 in CNS pathologies.
Spadin was previously described as a specific blocker of TREK-1 with a high affinity (IC 50 = 40–70 nM) ( Mazella et al., 2010 ; Djillani et al., 2017 , 2018 ). Spadin consists in 17-amino acid peptide synthesized from the propeptide (PE). PE is 44 amino acids generated from the maturation of sortilin by the cleavage of the prosortilin with furin ( Munck Petersen et al., 1999 ). Spadin binds with high affinities both TREK-1 and sortilin/neurotensin receptor 3 (NTSR3). Spadin by blocking TREK-1 generates mice with depression-resistance phenotype in several depression tests after only 4 days of treatment. Similarly to kcnk2 -/- mice, spadin enhances 5-HT neurotransmission in DRN serotonergic neurons ( Mazella et al., 2010 ). In in vitro experiments, spadin stimulates MAPK and PI3K pathways in time and concentration-dependent manner. Spadin at 100 nM increases the phosphorylation of ERK1/2 and Akt but does not affect the phosphorylation of mTOR, suggesting an original mechanism of action of spadin different from the other fast-acting antidepressant ketamine which was demonstrated to be mTOR-dependent ( Devader et al., 2015 ). Spadin has protective effects on neurons against staurosporine-induced Caspase-3 apoptosis through the specific activation of the PI3K pathway. Spadin increases transiently and after 8 h the mRNA expression of PSD-95 and synapsin, two markers of synaptogenesis in the brain. This increase is associated with a transient BDNF increase only after 5 h. Spadin increases also the proportion of mature spines in cortical neuronal culture. In vivo , daily spadin administration for 4 days increased mRNA expression of BDNF, PSD-95 and synapsin after only 7 days in the hippocampus. However, in the prefrontal cortex (PFC), only BDNF was enhanced after 3 weeks ( Devader et al., 2015 ).
The FST antidepressant activities of spadin disappear after 7 h after an acute treatment. To improve the bioavailability and the in vivo stability of spadin, retro-inverso analogs of spadin were designed and screened on the cell line stably expressing the human TREK-1 ( Veyssiere et al., 2015 ). Two analogs, 3 and 8, were identified. They prolong the antidepressant activity from 7 to 16 h in FST. However, due to some issues concerning in vitro toxicity of analog 3 and 8 at higher concentrations, other strategies were conducted in order to ameliorate the benefit/risk ratio. By shortening spadin sequence, a peptide PE 22-28 containing only 7 amino acids was identified as the shortest efficient molecule displaying an antidepressant activity ( Djillani et al., 2017 , 2018 ). The role of TREK-1 in mice was supported by other studies in human like STAR( ∗ )D which highlights a strong association between resistance to SSRIs and the existence of four single nucleotide polymorphisms (SNP) in kcnk2 gene ( Perlis et al., 2008 ). Another SNP, rs6686529 located in kcnk2 gene was found to be associated with major depression disorder and response to antidepressant treatment ( Liou et al., 2009 ). All these data highlight the key role of TREK-1 in depression and the need to design blockers of TREK-1 as promising original antidepressant ( Figure 3 ).
TREK-1 in Neuroprotection
Trek-1 in epileptogenesis.
TREK-1 is expressed in GABA-containing interneurons in the cortex and the hippocampus ( Hervieu et al., 2001 ). In vivo , It was reported that TREK-1 activators PUFA such as α-linolenic acid protect rats treated with the glutamate receptor agonist, kainic acid (KA) against seizures and hippocampal lesions ( Lauritzen et al., 2000 ). Moreover, PUFA neuroprotection was observed in another model of seizures using glutamatergic neurons. This effect was associated with the inhibition of the glutamatergic neurotransmission ( Lauritzen et al., 2000 ). TREK-1 deficient mice were shown to be more vulnerable to develop epileptic seizures triggered by KA and pentylenetetrazol (PTZ, GABA A receptor antagonist) ( Heurteaux et al., 2004 ). The expression of c-fos , a marker of neuronal excitability was increased in CA3 pyramidal neurons following treatment with KA ( Heurteaux et al., 2004 ). Linolenic acid and lysophosphatidylcholine (LPC) decrease KA-induced seizures in wild-type mice. However, no effect was observed in mice lacking TREK-1 channels ( Heurteaux et al., 2004 ). The protective effects of linolenic acid and LPC require the presence of TREK-1 channels. Status epilepticus (SE) is a persistent repeated seizure activity for more than 5 min. Development of TREK-1 mutant (TREK-M) produced a constitutively open TREK-1 channels that showed resistance to PKA and PKC downregulation. TREK-M was able to hyperpolarize the plasma membrane and decrease spontaneous firing of hippocampal neurons in culture ( Dey et al., 2014 ). In vivo administration of a recombinant adenoassociated virus (AAV)-mediated delivery of TREK-M in both entorhinal cortex and hippocampal CA3 regions, reduced by 50% the duration of SE in a mouse model of SE induced with lithium and pilocarpine. AAV-TREK-M prevented neuronal death in both entorhinal cortex and CA3 after injection ( Table 3 ; Dey et al., 2014 ). Since TREK-1 opening results in neuroprotection against epileptic episodes, blocking this AA-activated K 2P channel could in contrast be deleterious. Surprisingly, TREK-1 antagonist spadin does not enhance seizures induced by KA or PTZ when injected to mice. More interestingly, mice treated with spadin show more resistance to develop generalized convulsions and to induce death ( Moha Ou Maati et al., 2012 ; Djillani et al., 2018 ).
TREK-1 in ischemia
Using a model of global ischemia, results from transient bilateral occlusion of common carotid arteries in wild-type and knock-out TREK-1 mice showed that 74% of TREK -/- mice died 3 days after ischemia whereas only 34% died in wild-type group ( Heurteaux et al., 2004 ). Here again, linolenic acid or LPC pretreatment were unable to protect against ischemia in TREK-1 deficient mice in contrast to wild-type mice in which mice survival was significantly increased ( Heurteaux et al., 2004 ). Spinal cord ischemia is induced in mice by occluding both the aortic arch and left sub-clavian artery. It was reported that 75% of kcnk2 -/- mice died 3 h after 10 min ischemia compared to only 14% of wild-type mice 24 h after ischemia ( Heurteaux et al., 2004 ). Furthermore, surviving TREK-1 -/- mice developed severe hind limb paralysis. However, no neurological deficit was observed with wild-type mice ( Heurteaux et al., 2004 ). In addition to neurons, astrocytes were shown to play an important role in brain ischemia. TREK-1 contributes in maintaining the highly negative membrane potential in astrocytes which is crucial in controlling cell excitability ( Zhou et al., 2009 ). In cultured cortical astrocytes and in hippocampal slices, TREK-1 is widely expressed. Cortical and hippocampal TREK-1 channels were upregulated during astrogliosis following focal ischemia ( Wang et al., 2012 ). In hypoxic conditions, TREK-1 protein expression in astrocytes was also upregulated which increases glutamate clearance, suppressed the astrocytic marker S100β and block neuronal death ( Table 3 ; Wu et al., 2013 ). Glutamate is the main excitatory neurotransmitter in the brain. Astrocytes release glutamate following GPCR activation. DH Woo et al. demonstrate that TREK-1 channel is involved in the fast glutamate release which requires the interaction between the N-terminal domain of TREK-1 and the G βγ of the G αi PCR ( Woo et al., 2012 ).
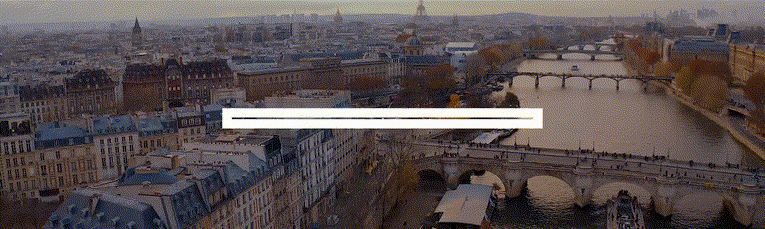
TREK-1 in general anesthesia
In general anesthesia, at clinical doses used for isoflurane, diethyl ether, halothane and chloroform, TREK-1 channels are activated ( Patel et al., 1999 ). TREK-1 channel activation implies the C-terminal moiety and leads to cell hyperpolarization, decreased action potential firing and neuroprotection. The laughing gas nitrous oxide and xenon mostly exert their anesthetic effects via antagonizing NMDA receptors since they provide analgesia, euphoria and neuroprotection. They do not potentiate GABA A receptors ( Gruss et al., 2004 ). Nitrous oxide and xenon were shown to open TREK-1 channels with Glu306 as a critical amino acid for the anesthetic activation of TREK-1 ( Gruss et al., 2004 ). Chloral hydrate is used in pediatrics in certain forms of epilepsy such as progressive myoclonus and refractory childhood epilepsies. Upon administration, Chloral hydrate is rapidly metabolized into its active metabolite 2,2,2-trichlorethanol (TCE) which is thought to be responsible for the chloral hydrate activity ( Harinath and Sikdar, 2004 ). TCE induces depression of the CNS by potentiating GABA A receptors and inhibiting NMDA, AMPA, and Kainate receptors. It was reported that TCE also activates TREK-1 and TRAAK channels and contributes to the central anesthetic effect ( Harinath and Sikdar, 2004 ). In vivo , mice lacking TREK-1 channels displayed a lower sensitivity to the most potent TREK-1 activators, chloroform and halothane and also to sevoflurane, desflurane and isoflurane ( Heurteaux et al., 2004 ). The threshold to provoke anesthesia and the doses required for the anesthetic action were higher. Phenobarbital, a general anesthetic that does not modulate TREK-1 channels, fails to reproduce these effects in kcnk2 -/- mice. Thus, the observed effects were due specifically to TREK channel absence. Recently, in vitro and in vivo preconditioning with sevoflurane was shown to provide neuroprotection through activation of TREK-1 channels ( Tong et al., 2014 ). Rats treated with the anesthetic sevoflurane after MCAO display neuroprotection which seems to be mediated by TREK-1 channels ( Table 3 ; Pan et al., 2017 ).
Role of TREK-1 in Post-stroke Depression
Given the neuroprotective role of TREK-1 channels in stroke and its involvement in the depression process. It makes sense to address the role of this K 2P channel in the physiopathology of post-stroke depression (PSD). Recently, several laboratories have started to investigate the potential role of TREK-1 channels in this pathology. PSD is a high prevalence neuropsychiatric disorder that takes place after stroke onset. It has been shown that TREK-1 channel expression levels were elevated in the hippocampus and the PFC in rat model of PSD ( Table 3 ; Lin et al., 2015 ). Noteworthy, TREK-1 channel up-regulation was suppressed following chronic treatment with the TREK-1 blocker and the SSRI, escitalopram. In this PSD rat model, the main actors causing PSD in human patients were reproduced. For example, the middle cerebral artery occlusion (MCAO) surgery was set up to mimic stroke and chronic mild stress (CMS) and isolation rearing to mimic stress and psychosocial conditions, respectively ( Lin et al., 2015 ).
TREK-1 in Pain Perception
TREK-1 channels are known to be modulated by heat. Those K 2P channels are gradually and reversibly opened by temperature. Noteworthy, TREK-1 channel opening is reversed by cAMP and prostaglandin E2, two sensitizers of peripheral and central thermoreceptors. TREK-1 blockade is due to the phosphorylation of Ser333 on its C-terminal end. The thermosensitive TREK-1 channel is highly expressed and localized in small dorsal root ganglion (DRG) neurons and in central hypothalamic neurons, areas that are highly involved in pain ( Maingret et al., 2000 ). Sixty percent of sensory neurons express TREK-1 and most of them are associated with substance P. More than 40% of DRG neurons that express TREK-1 channels also express the thermal nociceptor TRPV1 channels. In vivo , compared to wild type mice, mice lacking kcnk2 gene are highly sensitive to low-threshold thermal pain and mechanical stimuli ( Alloui et al., 2006 ). TREK-1 deficient mice showed an exacerbated focal inflammatory response after spinal cord injury (SCI). Furthermore, TREK-1 deficiency enhanced astrogliosis, neuronal apoptosis, demyelination and retarded motor recovery ( Fang et al., 2017 ). Nevertheless, in other reported studies, in the DRG, the microRNA miR-183 expression was decreased and TREK-1 expression was increased in neuropathic pain induced by chronic constriction of sciatic nerve (CCI) ( Table 3 ; Han et al., 2016 ; Shi et al., 2018 ). Intrathecal injection of miR-183 alleviated pain in rat model of CCI and downregulated TREK-1 expression ( Shi et al., 2018 ). Recently, it was reported that TREK-1 modulator riluzole prevents both sensory and motor deficits in neuropathic pain induced by the chemotherapy drug oxaliplatin ( Poupon et al., 2018 ). Nevertheless, conclusions drawn by this paper are not totally convincing because authors only consider riluzole as an activator and do not take into account its inhibitory effects. Finally, TREK-2 and TRAAK channels, the other members of TREK subfamily are characterized in the small sized DRG neurons of rats ( Viatchenko-Karpinski et al., 2018 ).
Peripheral Roles of TREK-1 Channels
Trek-1 in the heart.
In the heart, the stretch-activated K + channels (SAK) repolarize cell membrane and counterbalance the activity of stretch-activated Cation channels (SAC) which in contrast increase cation influx and cell depolarization. TREK-1 channel presents a serious candidate to form SAK in heart with the large conductance Ca 2+ -activated K + channel (BK Ca ) and K ATP channels ( Decher et al., 2017a ). In rat heart, all K 2P channel genes were detected in at least one heart chamber with a prevalence of TWIK-2, TASK-1 and TREK-1 expression. TREK-1 channel was highly expressed in the right ventricle ( Liu and Saint, 2004 ). In rat neonatal atrial myocytes, using single channel inside-out patch, TREK-1-like current was activated by AA and internal acidosis ( Kim and Clapham, 1989 ). This TREK-1-like current was shown to be reversibly activated by volatile anesthetics such as chloroform, halothane and isoflurane. It was also downregulated by cAMP analogs and β-adrenergic receptor agonists ( Terrenoire et al., 2001 ). SAK current shared identical biophysical properties with the recombinant TREK-1 current such as the single channel conductance, no voltage-dependency and sensitivity to volatile anesthetics. In the ventricular cardiomyocytes, two variants of TREK-1 were found, large conductance and small conductance with (132 ± 5 pS) and (41 ± 5 pS), respectively, at positive potentials ( Xian Tao et al., 2006 ). The low-conductance TREK-1 variant was opened by mechanical stretch, internal acidification and AA. The biophysical properties of the two TREK-1 channels were similar to those displayed by the recombinant TREK-1 channels expressed in HEK293 cells. Using cardiac specific TREK-1 deficient mice, it was proposed that TREK-1 plays an essential role in sinoatrial node cell excitability ( Unudurthi et al., 2016 ). Recently, a heterozygous point mutation in the selectivity filter of TREK-1 channel was identified in patients diagnosed with right ventricular outflow tract (RVOT) tachycardia ( Decher et al., 2017b ). More interestingly, TREK-M showed a hypersensitivity to stretch and permeability to sodium ions ( Decher et al., 2017b ; Figure 3 ).
Role of TREK-1 in Other Tissues
In addition to the central roles of TREK-1 channels in the brain, it was thoroughly rational to think that modulating TREK-1 channels could play a peripheral role as this K 2P channel is expressed in number of tissues such as pancreas, prostate and smooth muscle cells ( Medhurst et al., 2001 ). The pancreas and particularly the insulin secreting β-cells constitute one of the main targets of the peripheral tissues where TREK-1 and other K 2P channels play an important role in glucose homeostasis ( Kang et al., 2004 ; Mazella et al., 2010 ; Dadi et al., 2014 ; Vierra et al., 2015 ). Given the importance of TREK-1 channel as a key modulator of plasma membrane potential in addition to its regulation by various physical and chemical stimuli, its role in the glucose homeostasis has been investigated using spadin ( Hivelin et al., 2016 ). Spadin inhibits TREK-1 channels in the pancreatic β-cell line β-TC3 ( Mazella et al., 2010 ). It is known that the regulation of insulin secretion by pancreatic β-cell is fine-tuned by a variety of hormones such as glucagon like-peptide-1 (GLP-1), leptin, estrogen, melatonin and growth hormone ( Fu et al., 2013 ). It is well established that ATP-sensitive potassium channels (K ATP ) play a critical role in maintaining glucose homeostasis ( Mctaggart et al., 2010 ). K ATP channels serve as a metabolic sensor for the pancreatic β-cell. When glucose levels increase, K ATP channels close, inducing a membrane depolarization resulting in the opening of Ca 2+ channels as well as the secretion of numerous hormones and amplification of the effects of glucose and other secretagogues such as GLP-1 ( Mctaggart et al., 2010 ). TREK-1 blockade by spadin induces insulin secretion only upon glucose stimulation. However, in contrast to incretin hormones, spadin induces Ca 2+ increase and insulin release through an original PKA-independent mechanism ( Hivelin et al., 2016 ). TREK-1 channels are inhibited as well by the PKA phosphorylation of the Ser333 at the C-terminal moiety of the channel ( Murbartián et al., 2005 ), which is the consequence of the activation of different G-protein-coupled receptors at the plasma membrane. By binding to their GLP-1 receptors, GLP-1 and its analogs such as exendin-4 are able to trigger cAMP synthesis and thus induce PKA activation leading to insulin secretion ( Göke et al., 1993 ). Then, insulin release by β-cells is potentiated by TREK-1 closing following PKA phosphorylation. Spadin or its analogs could substitute to incretin hormone actions and could propose a therapeutic alternative in case of inefficacy of antidiabetic treatment using GLP-1 mimetics. Among the other K 2P channels, the mechano-sensitive K 2P channel TREK-1 is predominately expressed in the human detrusor channel ( Andersson and Arner, 2004 ). Recently, a study assessing the relationship between six SNP and the occurrence of overactive lower urinary tract symptoms (LUTS), revealed a strong association between TREK-1 polymorphisms (rs758937019-CT genotype and rs758937019-T allele) and overactive LUTS in human ( Nedumaran et al., 2019 ).
Conclusion and Perspectives
Since their cloning 20 years ago, the physiological importance of TREK-1 channels has continued to grow ( Figure 3 ). Today, TREK-1 channels have been shown to be important and their presence is essential in a number of physiopathological processes. Their involvement in these different processes demonstrate the necessity to design pharmacological modulators, activators or inhibitors, of these channels to correct any TREK-1-related dysfunctions.
Despites a number of studies and many molecule screenings, only few putative new drugs were identified. The activators belonging to the ML and BL series show interesting results. However, they display lower affinities (in the micromolar range) and they are not specific for TREK-1 channels as they open other K 2P channels. TREK-1 openers are needed in pathologies such as ischemia, epilepsy and pain. The challenge is to improve their affinity, specificity and ability to cross the blood-brain barrier. The new ML and BL series of TREK- activators have not yet been tested in pathologies such epilepsy and ischemia. It would be interesting to investigate any neuroprotection effects of these molecules in rodent models of epilepsy or ischemia. In the same time, their specificity should be improved to avoid any off-target effects resulting from modulating other K 2P channels.
As inhibitors, the most promising molecules are spadin and its analogs. These peptides are very specific in the K 2P family and display very high affinities, in the picomolar range for the shorter analogs ( Djillani et al., 2017 ). Depression is a complex mood disorder where different actors are involved. TREK-1 is definitely one of the most promising ion channel that plays a key role in the neurobiology of depression. TREK-1 blockers such as spadin and short analogs display a huge therapeutic value over the classical antidepressants prescribed nowadays. They are fast-acting with highly affinity and specificity for TREK-1. More interestingly, they cross the blood-brain barrier which facilitates their further optimization and development as antidepressant drugs useful in clinic.
In the future, in addition to looking for new TREK-1 modulators to treat neuropsychiatric disorders, it is relevant to study the role of TREK-1 channels in peripheral tissues and understand their involvement in physiopathology. An emerging area where TREK-1 is displaying a growing interest is the heart. TREK-1 expression has been shown to change in cardiac hypertrophy ( Wang et al., 2013 ). Furthermore, a TREK-M was found to change channel permeability to sodium and cause ventricular tachycardia ( Decher et al., 2017b ).
To conclude, the wide distribution of TREK-1 in human body, the diverse stimuli and regulations that it could respond to, and the pleiotropic roles it could play in physiology and physiopathology, make TREK-1 a promising target to explore in depth but at the same time very challenging to design modulators that are tissue-specific.
Author Contributions
AD wrote the manuscript. MB, CH, and JM corrected it.
Conflict of Interest Statement
The authors declare that the research was conducted in the absence of any commercial or financial relationships that could be construed as a potential conflict of interest.
- Alloui A., Zimmermann K., Mamet J., Duprat F., Noel J., Chemin J., et al. (2006). TREK-1, a K+ channel involved in polymodal pain perception. EMBO J. 25 2368–2376. 10.1038/sj.emboj.7601116 [ PMC free article ] [ PubMed ] [ CrossRef ] [ Google Scholar ]
- Andersson K. E., Arner A. (2004). Urinary bladder contraction and relaxation: physiology and pathophysiology. Physiol. Rev. 84 935–986. 10.1152/physrev.00038.2003 [ PubMed ] [ CrossRef ] [ Google Scholar ]
- Bagriantsev S. N., Ang K. H., Gallardo-Godoy A., Clark K. A., Arkin M. R., Renslo A. R., et al. (2013). A high-throughput functional screen identifies small molecule regulators of temperature- and mechano-sensitive K2P channels. ACS Chem. Biol. 8 1841–1851. 10.1021/cb400289x [ PMC free article ] [ PubMed ] [ CrossRef ] [ Google Scholar ]
- Barcia G., Fleming M. R., Deligniere A., Gazula V. R., Brown M. R., Langouet M., et al. (2012). De novo gain-of-function KCNT1 channel mutations cause malignant migrating partial seizures of infancy. Nat. Genet. 44 1255–1259. 10.1038/ng.2441 [ PMC free article ] [ PubMed ] [ CrossRef ] [ Google Scholar ]
- Barel O., Shalev S. A., Ofir R., Cohen A., Zlotogora J., Shorer Z., et al. (2008). Maternally inherited Birk Barel mental retardation dysmorphism syndrome caused by a mutation in the genomically imprinted potassium channel KCNK9. Am. J. Hum. Genet. 83 193–199. 10.1016/j.ajhg.2008.07.010 [ PMC free article ] [ PubMed ] [ CrossRef ] [ Google Scholar ]
- Bauer C. K., Calligari P., Radio F. C., Caputo V., Dentici M. L., Falah N., et al. (2018). Mutations in KCNK4 that affect gating cause a recognizable neurodevelopmental syndrome. Am. J. Hum. Genet. 103 621–630. 10.1016/j.ajhg.2018.09.001 [ PMC free article ] [ PubMed ] [ CrossRef ] [ Google Scholar ]
- Benatar M. (2000). Neurological potassium channelopathies. QJM 93 787–797. 10.1093/qjmed/93.12.787 [ PubMed ] [ CrossRef ] [ Google Scholar ]
- Blin S., Ben Soussia I., Kim E. J., Brau F., Kang D., Lesage F., et al. (2016). Mixing and matching TREK/TRAAK subunits generate heterodimeric K2P channels with unique properties. Proc. Natl. Acad. Sci. U.S.A. 113 4200–4205. 10.1073/pnas.1522748113 [ PMC free article ] [ PubMed ] [ CrossRef ] [ Google Scholar ]
- Brohawn S. G. (2015). How ion channels sense mechanical force: insights from mechanosensitive K2P channels TRAAK, TREK1, and TREK2. Ann. N. Y. Acad. Sci. 1352 20–32. 10.1111/nyas.12874 [ PubMed ] [ CrossRef ] [ Google Scholar ]
- Brohawn S. G., Su Z., Mackinnon R. (2014). Mechanosensitivity is mediated directly by the lipid membrane in TRAAK and TREK1 K+ channels. Proc. Natl. Acad. Sci. U.S.A. 111 3614–3619. 10.1073/pnas.1320768111 [ PMC free article ] [ PubMed ] [ CrossRef ] [ Google Scholar ]
- Brown D. A., Passmore G. M. (2009). Neural KCNQ (Kv7) channels. Br. J. Pharmacol. 156 1185–1195. 10.1111/j.1476-5381.2009.00111.x [ PMC free article ] [ PubMed ] [ CrossRef ] [ Google Scholar ]
- Busquet P., Nguyen N. K., Schmid E., Tanimoto N., Seeliger M. W., Ben-Yosef T., et al. (2010). CaV1.3 L-type Ca2+ channels modulate depression-like behaviour in mice independent of deaf phenotype. Int. J. Neuropsychopharmacol. 13 499–513. 10.1017/S1461145709990368 [ PubMed ] [ CrossRef ] [ Google Scholar ]
- Chemin J., Patel A. J., Duprat F., Lauritzen I., Lazdunski M., Honore E. (2005). A phospholipid sensor controls mechanogating of the K+ channel TREK-1. EMBO J. 24 44–53. 10.1038/sj.emboj.7600494 [ PMC free article ] [ PubMed ] [ CrossRef ] [ Google Scholar ]
- Chen C., Wang L., Rong X., Wang W., Wang X. (2015). Effects of fluoxetine on protein expression of potassium ion channels in the brain of chronic mild stress rats. Acta Pharm. Sin. B 5 55–61. 10.1016/j.apsb.2014.12.004 [ PMC free article ] [ PubMed ] [ CrossRef ] [ Google Scholar ]
- Cheramy A., Barbeito L., Godeheu G., Glowinski J. (1992). Riluzole inhibits the release of glutamate in the caudate nucleus of the cat in vivo. Neurosci. Lett. 147 209–212. 10.1016/0304-3940(92)90597-Z [ PubMed ] [ CrossRef ] [ Google Scholar ]
- Comoglio Y., Levitz J., Kienzler M. A., Lesage F., Isacoff E. Y., Sandoz G. (2014). Phospholipase D2 specifically regulates TREK potassium channels via direct interaction and local production of phosphatidic acid. Proc. Natl. Acad. Sci. U.S.A. 111 13547–13552. 10.1073/pnas.1407160111 [ PMC free article ] [ PubMed ] [ CrossRef ] [ Google Scholar ]
- Dadi P. K., Vierra N. C., Jacobson D. A. (2014). Pancreatic β-cell-specific ablation of TASK-1 channels augments glucose-stimulated calcium entry and insulin secretion, improving glucose tolerance. Endocrinology 155 3757–3768. 10.1210/en.2013-2051 [ PMC free article ] [ PubMed ] [ CrossRef ] [ Google Scholar ]
- Decher N., Kiper A. K., Rinne S. (2017a). Stretch-activated potassium currents in the heart: focus on TREK-1 and arrhythmias. Prog. Biophys. Mol. Biol. 130 223–232. 10.1016/j.pbiomolbio.2017.05.005 [ PubMed ] [ CrossRef ] [ Google Scholar ]
- Decher N., Ortiz-Bonnin B., Friedrich C., Schewe M., Kiper A. K., Rinne S., et al. (2017b). Sodium permeable and “hypersensitive” TREK-1 channels cause ventricular tachycardia. EMBO Mol. Med. 9 403–414. 10.15252/emmm.201606690 [ PMC free article ] [ PubMed ] [ CrossRef ] [ Google Scholar ]
- Devader C., Khayachi A., Veyssiere J., Moha Ou Maati H., Roulot M., Moreno S., et al. (2015). In vitro and in vivo regulation of synaptogenesis by the novel antidepressant spadin. Br. J. Pharmacol. 172 2604–2617. 10.1111/bph.13083 [ PMC free article ] [ PubMed ] [ CrossRef ] [ Google Scholar ]
- Dey D., Eckle V. S., Vitko I., Sullivan K. A., Lasiecka Z. M., Winckler B., et al. (2014). A potassium leak channel silences hyperactive neurons and ameliorates status epilepticus. Epilepsia 55 203–213. 10.1111/epi.12472 [ PMC free article ] [ PubMed ] [ CrossRef ] [ Google Scholar ]
- Djillani A., Pietri M., Mazella J., Heurteaux C., Borsotto M. (2018). Fighting against depression with TREK-1 blockers: past and future. A focus on spadin. Pharmacol. Ther. 194 185–198. 10.1016/j.pharmthera.2018.10.003 [ PubMed ] [ CrossRef ] [ Google Scholar ]
- Djillani A., Pietri M., Moreno S., Heurteaux C., Mazella J., Borsotto M. (2017). Shortened spadin analogs display better TREK-1 inhibition, in vivo stability and antidepressant activity. Front. Pharmacol. 8 : 643 . 10.3389/fphar.2017.00643 [ PMC free article ] [ PubMed ] [ CrossRef ] [ Google Scholar ]
- Du W., Bautista J. F., Yang H., Diez-Sampedro A., You S. A., Wang L., et al. (2005). Calcium-sensitive potassium channelopathy in human epilepsy and paroxysmal movement disorder. Nat. Genet. 37 733–738. 10.1038/ng1585 [ PubMed ] [ CrossRef ] [ Google Scholar ]
- Duprat F., Lesage F., Patel A. J., Fink M., Romey G., Lazdunski M. (2000). The neuroprotective agent riluzole activates the two P domain K(+) channels TREK-1 and TRAAK. Mol. Pharmacol. 57 906–912. [ PubMed ] [ Google Scholar ]
- Esseltine J. L., Scott J. D. (2013). AKAP signaling complexes: pointing towards the next generation of therapeutic targets? Trends Pharmacol. Sci. 34 648–655. 10.1016/j.tips.2013.10.005 [ PMC free article ] [ PubMed ] [ CrossRef ] [ Google Scholar ]
- Fang Y., Huang X., Wan Y., Tian H., Tian Y., Wang W., et al. (2017). Deficiency of TREK-1 potassium channel exacerbates secondary injury following spinal cord injury in mice. J. Neurochem. 141 236–246. 10.1111/jnc.13980 [ PubMed ] [ CrossRef ] [ Google Scholar ]
- Fink M., Duprat F., Lesage F., Reyes R., Romey G., Heurteaux C., et al. (1996). Cloning, functional expression and brain localization of a novel unconventional outward rectifier K+ channel. EMBO J. 15 6854–6862. 10.1002/j.1460-2075.1996.tb01077.x [ PMC free article ] [ PubMed ] [ CrossRef ] [ Google Scholar ]
- Fu Z., Gilbert E. R., Liu D. (2013). Regulation of insulin synthesis and secretion and pancreatic Beta-cell dysfunction in diabetes. Curr. Diabetes Rev. 9 25–53. 10.2174/1573399811309010025 [ PMC free article ] [ PubMed ] [ CrossRef ] [ Google Scholar ]
- Gloyn A. L., Pearson E. R., Antcliff J. F., Proks P., Bruining G. J., Slingerland A. S., et al. (2004). Activating mutations in the gene encoding the ATP-sensitive potassium-channel subunit Kir6.2 and permanent neonatal diabetes. N. Engl. J. Med. 350 1838–1849. 10.1056/NEJMoa032922 [ PubMed ] [ CrossRef ] [ Google Scholar ]
- Göke R., Fehmann H. C., Linn T., Schmidt H., Krause M., Eng J., et al. (1993). Exendin-4 is a high potency agonist and truncated exendin-(9–39)-amide an antagonist at the glucagon-like peptide 1-(7–36)-amide receptor of insulin-secreting β-cells. J. Biol. Chem. 268 19650–19655. [ PubMed ] [ Google Scholar ]
- Gomez-Navarro N., Miller E. A. (2016). COP-coated vesicles. Curr. Biol. 26 R54–R57. 10.1016/j.cub.2015.12.017 [ PubMed ] [ CrossRef ] [ Google Scholar ]
- Gruss M., Bushell T. J., Bright D. P., Lieb W. R., Mathie A., Franks N. P. (2004). Two-pore-domain K+ channels are a novel target for the anesthetic gases xenon, nitrous oxide, and cyclopropane. Mol. Pharmacol. 65 443–452. 10.1124/mol.65.2.443 [ PubMed ] [ CrossRef ] [ Google Scholar ]
- Han H. J., Lee S. W., Kim G. T., Kim E. J., Kwon B., Kang D., et al. (2016). Enhanced Expression of TREK-1 Is related with chronic constriction injury of neuropathic pain mouse model in dorsal root ganglion. Biomol. Ther. 24 252–259. 10.4062/biomolther.2016.038 [ PMC free article ] [ PubMed ] [ CrossRef ] [ Google Scholar ]
- Harinath S., Sikdar S. K. (2004). Trichloroethanol enhances the activity of recombinant human TREK-1 and TRAAK channels. Neuropharmacology 46 750–760. 10.1016/j.neuropharm.2003.11.023 [ PubMed ] [ CrossRef ] [ Google Scholar ]
- Hervieu G. J., Cluderay J. E., Gray C. W., Green P. J., Ranson J. L., Randall A. D., et al. (2001). Distribution and expression of TREK-1, a two-pore-domain potassium channel, in the adult rat CNS. Neuroscience 103 899–919. 10.1016/S0306-4522(01)00030-6 [ PubMed ] [ CrossRef ] [ Google Scholar ]
- Heurteaux C., Guy N., Laigle C., Blondeau N., Duprat F., Mazzuca M., et al. (2004). TREK-1, a K+ channel involved in neuroprotection and general anesthesia. EMBO J. 23 2684–2695. 10.1038/sj.emboj.7600234 [ PMC free article ] [ PubMed ] [ CrossRef ] [ Google Scholar ]
- Heurteaux C., Lucas G., Guy N., El Yacoubi M., Thummler S., Peng X. D., et al. (2006). Deletion of the background potassium channel TREK-1 results in a depression-resistant phenotype. Nat. Neurosci. 9 1134–1141. 10.1038/nn1749 [ PubMed ] [ CrossRef ] [ Google Scholar ]
- Hirano K., Kimura R., Sugimoto Y., Yamada J., Uchida S., Kato Y., et al. (2005). Relationship between brain serotonin transporter binding, plasma concentration and behavioural effect of selective serotonin reuptake inhibitors. Br. J. Pharmacol. 144 695–702. 10.1038/sj.bjp.0706108 [ PMC free article ] [ PubMed ] [ CrossRef ] [ Google Scholar ]
- Hivelin C., Béraud-Dufour S., Devader C., Abderrahmani A., Moreno S., Moha Ou Maati H., et al. (2016). Potentiation of calcium influx and insulin secretion in pancreatic beta cell by the specific TREK-1 blocker spadin. J. Diabetes Res. 2016 : 3142175 . 10.1155/2016/3142175 [ PMC free article ] [ PubMed ] [ CrossRef ] [ Google Scholar ]
- Honore E. (2007). The neuronal background K2P channels: focus on TREK1. Nat. Rev. Neurosci. 8 251–261. 10.1038/nrn2117 [ PubMed ] [ CrossRef ] [ Google Scholar ]
- Hubert J. P., Delumeau J. C., Glowinski J., Premont J., Doble A. (1994). Antagonism by riluzole of entry of calcium evoked by NMDA and veratridine in rat cultured granule cells: evidence for a dual mechanism of action. Br. J. Pharmacol. 113 261–267. 10.1111/j.1476-5381.1994.tb16203.x [ PMC free article ] [ PubMed ] [ CrossRef ] [ Google Scholar ]
- Hwang E. M., Kim E., Yarishkin O., Woo D. H., Han K. S., Park N., et al. (2014). A disulphide-linked heterodimer of TWIK-1 and TREK-1 mediates passive conductance in astrocytes. Nat. Commun. 5 : 3227 . 10.1038/ncomms4227 [ PubMed ] [ CrossRef ] [ Google Scholar ]
- Ji X. C., Zhao W. H., Cao D. X., Shi Q. Q., Wang X. L. (2011). Novel neuroprotectant chiral 3-n-butylphthalide inhibits tandem-pore-domain potassium channel TREK-1. Acta Pharmacol. Sin. 32 182–187. 10.1038/aps.2010.210 [ PMC free article ] [ PubMed ] [ CrossRef ] [ Google Scholar ]
- Kang D., Choe C., Kim D. (2004). Functional expression of TREK-2 in insulin-secreting MIN6 cells. Biochem. Biophys. Res. Commun. 323 323–331. 10.1016/j.bbrc.2004.08.089 [ PubMed ] [ CrossRef ] [ Google Scholar ]
- Kennard L. E., Chumbley J. R., Ranatunga K. M., Armstrong S. J., Veale E. L., Mathie A. (2005). Inhibition of the human two-pore domain potassium channel, TREK-1, by fluoxetine and its metabolite norfluoxetine. Br. J. Pharmacol. 144 821–829. 10.1038/sj.bjp.0706068 [ PMC free article ] [ PubMed ] [ CrossRef ] [ Google Scholar ]
- Kim D., Clapham D. E. (1989). Potassium channels in cardiac cells activated by arachidonic acid and phospholipids. Science 244 1174–1176. 10.1126/science.2727703 [ PubMed ] [ CrossRef ] [ Google Scholar ]
- Kim E., Hwang E. M., Yarishkin O., Yoo J. C., Kim D., Park N., et al. (2010). Enhancement of TREK1 channel surface expression by protein-protein interaction with beta-COP. Biochem. Biophys. Res. Commun. 395 244–250. 10.1016/j.bbrc.2010.03.171 [ PubMed ] [ CrossRef ] [ Google Scholar ]
- Kim E. J., Lee D. K., Hong S. G., Han J., Kang D. (2017). Activation of TREK-1, but Not TREK-2, channel by mood stabilizers. Int. J. Mol. Sci. 18 : E2460 . 10.3390/ijms18112460 [ PMC free article ] [ PubMed ] [ CrossRef ] [ Google Scholar ]
- Kindler C. H., Yost C. S., Gray A. T. (1999). Local anesthetic inhibition of baseline potassium channels with two pore domains in tandem. Anesthesiology 90 1092–1102. 10.1097/00000542-199904000-00024 [ PubMed ] [ CrossRef ] [ Google Scholar ]
- Lafreniere R. G., Cader M. Z., Poulin J. F., Andres-Enguix I., Simoneau M., Gupta N., et al. (2010). A dominant-negative mutation in the TRESK potassium channel is linked to familial migraine with aura. Nat. Med. 16 1157–1160. 10.1038/nm.2216 [ PubMed ] [ CrossRef ] [ Google Scholar ]
- Lauritzen I., Blondeau N., Heurteaux C., Widmann C., Romey G., Lazdunski M. (2000). Polyunsaturated fatty acids are potent neuroprotectors. EMBO J. 19 1784–1793. 10.1093/emboj/19.8.1784 [ PMC free article ] [ PubMed ] [ CrossRef ] [ Google Scholar ]
- Lei Q., Pan X. Q., Chang S., Malkowicz S. B., Guzzo T. J., Malykhina A. P. (2014). Response of the human detrusor to stretch is regulated by TREK-1, a two-pore-domain (K2P) mechano-gated potassium channel. J. Physiol. 592 3013–3030. 10.1113/jphysiol.2014.271718 [ PMC free article ] [ PubMed ] [ CrossRef ] [ Google Scholar ]
- Lesage F., Guillemare E., Fink M., Duprat F., Lazdunski M., Romey G., et al. (1996). TWIK-1, a ubiquitous human weakly inward rectifying K+ channel with a novel structure. EMBO J. 15 1004–1011. 10.1002/j.1460-2075.1996.tb00437.x [ PMC free article ] [ PubMed ] [ CrossRef ] [ Google Scholar ]
- Lesage F., Lazdunski M. (2000). Molecular and functional properties of two-pore-domain potassium channels. Am. J. Physiol. Renal Physiol. 279 F793–F801. 10.1152/ajprenal.2000.279.5.F793 [ PubMed ] [ CrossRef ] [ Google Scholar ]
- Levitz J., Royal P., Comoglio Y., Wdziekonski B., Schaub S., Clemens D. M., et al. (2016). Heterodimerization within the TREK channel subfamily produces a diverse family of highly regulated potassium channels. Proc. Natl. Acad. Sci. U.S.A. 113 4194–4199. 10.1073/pnas.1522459113 [ PMC free article ] [ PubMed ] [ CrossRef ] [ Google Scholar ]
- Lin D. H., Zhang X. R., Ye D. Q., Xi G. J., Hui J. J., Liu S. S., et al. (2015). The role of the two-pore domain potassium channel TREK-1 in the therapeutic effects of escitalopram in a rat model of poststroke depression. CNS Neurosci. Ther. 21 504–512. 10.1111/cns.12384 [ PMC free article ] [ PubMed ] [ CrossRef ] [ Google Scholar ]
- Liou Y. J., Chen T. J., Tsai S. J., Yu Y. W., Cheng C. Y., Hong C. J. (2009). Support for the involvement of the KCNK2 gene in major depressive disorder and response to antidepressant treatment. Pharmacogenet. Genomics 19 735–741. 10.1097/FPC.0b013e32832cbe61 [ PubMed ] [ CrossRef ] [ Google Scholar ]
- Liu H., Enyeart J. A., Enyeart J. J. (2007). Potent inhibition of native TREK-1 K+ channels by selected dihydropyridine Ca2+ channel antagonists. J. Pharmacol. Exp. Ther. 323 39–48. 10.1124/jpet.107.125245 [ PubMed ] [ CrossRef ] [ Google Scholar ]
- Liu W., Saint D. A. (2004). Heterogeneous expression of tandem-pore K+ channel genes in adult and embryonic rat heart quantified by real-time polymerase chain reaction. Clin. Exp. Pharmacol. Physiol. 31 174–178. 10.1111/j.1440-1681.2004.03964.x [ PubMed ] [ CrossRef ] [ Google Scholar ]
- Lolicato M., Arrigoni C., Mori T., Sekioka Y., Bryant C., Clark K. A., et al. (2017). K2P2.1 (TREK-1)-activator complexes reveal a cryptic selectivity filter binding site. Nature 547 364–368. 10.1038/nature22988 [ PMC free article ] [ PubMed ] [ CrossRef ] [ Google Scholar ]
- Lopes C. M., Rohacs T., Czirjak G., Balla T., Enyedi P., Logothetis D. E. (2005). PIP2 hydrolysis underlies agonist-induced inhibition and regulates voltage gating of two-pore domain K+ channels. J. Physiol. 564 117–129. 10.1113/jphysiol.2004.081935 [ PMC free article ] [ PubMed ] [ CrossRef ] [ Google Scholar ]
- Loucif A. J. C., Saintot P. P., Liu J., Antonio B. M., Zellmer S. G., Yoger K., et al. (2018). GI-530159, a novel, selective, mechanosensitive two-pore-domain potassium (K2P ) channel opener, reduces rat dorsal root ganglion neuron excitability. Br. J. Pharmacol. 175 2272–2283. 10.1111/bph.14098 [ PMC free article ] [ PubMed ] [ CrossRef ] [ Google Scholar ]
- Maingret F., Honore E., Lazdunski M., Patel A. J. (2002). Molecular basis of the voltage-dependent gating of TREK-1, a mechano-sensitive K(+) channel. Biochem. Biophys. Res. Commun. 292 339–346. 10.1006/bbrc.2002.6674 [ PubMed ] [ CrossRef ] [ Google Scholar ]
- Maingret F., Lauritzen I., Patel A. J., Heurteaux C., Reyes R., Lesage F., et al. (2000). TREK-1 is a heat-activated background K(+) channel. EMBO J. 19 2483–2491. 10.1093/emboj/19.11.2483 [ PMC free article ] [ PubMed ] [ CrossRef ] [ Google Scholar ]
- Maingret F., Patel A. J., Lesage F., Lazdunski M., Honore E. (1999). Mechano- or acid stimulation, two interactive modes of activation of the TREK-1 potassium channel. J. Biol. Chem. 274 26691–26696. 10.1074/jbc.274.38.26691 [ PubMed ] [ CrossRef ] [ Google Scholar ]
- Martin D., Thompson M. A., Nadler J. V. (1993). The neuroprotective agent riluzole inhibits release of glutamate and aspartate from slices of hippocampal area CA1. Eur. J. Pharmacol. 250 473–476. 10.1016/0014-2999(93)90037-I [ PubMed ] [ CrossRef ] [ Google Scholar ]
- Mazella J., Petrault O., Lucas G., Deval E., Beraud-Dufour S., Gandin C., et al. (2010). Spadin, a sortilin-derived peptide, targeting rodent TREK-1 channels: a new concept in the antidepressant drug design. PLoS Biol. 8 : e1000355 . 10.1371/journal.pbio.1000355 [ PMC free article ] [ PubMed ] [ CrossRef ] [ Google Scholar ]
- Mazella J., Zsürger N., Navarro V., Chabry J., Kaghad M., Caput D., et al. (1998). The 100-kDa neurotensin receptor is gp95/sortilin, a non-G-protein-coupled receptor. J. Biol. Chem. 273 26273–26276. 10.1074/jbc.273.41.26273 [ PubMed ] [ CrossRef ] [ Google Scholar ]
- Mcclenaghan C., Schewe M., Aryal P., Carpenter E. P., Baukrowitz T., Tucker S. J. (2016). Polymodal activation of the TREK-2 K2P channel produces structurally distinct open states. J. Gen. Physiol. 147 497–505. 10.1085/jgp.201611601 [ PMC free article ] [ PubMed ] [ CrossRef ] [ Google Scholar ]
- McTaggart J. S., Clark R. H., Ashcroft F. M. (2010). The role of the K ATP channel in glucose homeostasis in health and disease: more than meets the islet. J. Physiol. 588 3201–3209. 10.1113/jphysiol.2010.191767 [ PMC free article ] [ PubMed ] [ CrossRef ] [ Google Scholar ]
- Medhurst A. D., Rennie G., Chapman C. G., Meadows H., Duckworth M. D., Kelsell R. E., et al. (2001). Distribution analysis of human two pore domain potassium channels in tissues of the central nervous system and periphery. Brain Res. Mol. Brain Res. 86 101–114. 10.1016/S0169-328X(00)00263-1 [ PubMed ] [ CrossRef ] [ Google Scholar ]
- Moha Ou Maati H., Veyssiere J., Labbal F., Coppola T., Gandin C., Widmann C., et al. (2012). Spadin as a new antidepressant: absence of TREK-1-related side effects. Neuropharmacology 62 278–288. 10.1016/j.neuropharm.2011.07.019 [ PubMed ] [ CrossRef ] [ Google Scholar ]
- Munck Petersen C., Nielsen M. S., Jacobsen C., Tauris J., Jacobsen L., Gliemann J., et al. (1999). Propeptide cleavage conditions sortilin/neurotensin receptor-3 for ligand binding. EMBO J. 18 595–604. 10.1093/emboj/18.3.595 [ PMC free article ] [ PubMed ] [ CrossRef ] [ Google Scholar ]
- Murbartián J., Lei Q., Sando J. J., Bayliss D. A. (2005). Sequential phosphorylation mediates receptor- and kinase-induced inhibition of TREK-1 background potassium channels. J. Biol. Chem. 280 30175–30184. 10.1074/jbc.M503862200 [ PubMed ] [ CrossRef ] [ Google Scholar ]
- Nedumaran B., Pineda R. H., Rudra P., Lee S., Malykhina A. P. (2019). Association of genetic polymorphisms in the pore domains of mechano-gated TREK-1 channel with overactive lower urinary tract symptoms in humans. Neurourol. Urodyn. 38 144–150. 10.1002/nau.23862 [ PubMed ] [ CrossRef ] [ Google Scholar ]
- Pan L., Yang F., Lu C., Jia C., Wang Q., Zeng K. (2017). Effects of sevoflurane on rats with ischemic brain injury and the role of the TREK-1 channel. Exp. Ther. Med. 14 2937–2942. 10.3892/etm.2017.4906 [ PMC free article ] [ PubMed ] [ CrossRef ] [ Google Scholar ]
- Patel A. J., Honore E., Lesage F., Fink M., Romey G., Lazdunski M. (1999). Inhalational anesthetics activate two-pore-domain background K+ channels. Nat. Neurosci. 2 422–426. 10.1038/8084 [ PubMed ] [ CrossRef ] [ Google Scholar ]
- Patel A. J., Honore E., Maingret F., Lesage F., Fink M., Duprat F., et al. (1998). A mammalian two pore domain mechano-gated S-like K+ channel. EMBO J. 17 4283–4290. 10.1093/emboj/17.15.4283 [ PMC free article ] [ PubMed ] [ CrossRef ] [ Google Scholar ]
- Perlis R. H., Moorjani P., Fagerness J., Purcell S., Trivedi M. H., Fava M., et al. (2008). Pharmacogenetic analysis of genes implicated in rodent models of antidepressant response: association of TREK1 and treatment resistance in the STAR( ∗ )D study. Neuropsychopharmacology 33 2810–2819. 10.1038/npp.2008.6 [ PMC free article ] [ PubMed ] [ CrossRef ] [ Google Scholar ]
- Petersen C. M., Nielsen M. S., Nykjær A., Jacobsen L., Tommerup N., Rasmussen H. H., et al. (1997). Molecular identification of a novel candidate sorting receptor purified from human brain by receptor-associated protein affinity chromatography. J. Biol. Chem. 272 3599–3605. 10.1074/jbc.272.6.3599 [ PubMed ] [ CrossRef ] [ Google Scholar ]
- Pietrobon D. (2002). Calcium channels and channelopathies of the central nervous system. Mol. Neurobiol. 25 31–50. 10.1385/MN:25:1:031 [ PubMed ] [ CrossRef ] [ Google Scholar ]
- Pope L., Arrigoni C., Lou H., Bryant C., Gallardo-Godoy A., Renslo A. R., et al. (2018). Protein and Chemical Determinants of BL-1249 Action and Selectivity for K2P Channels. ACS Chem. Neurosci. 10.1021/acschemneuro.8b00337 [Epub ahead of print]. [ PMC free article ] [ PubMed ] [ CrossRef ] [ Google Scholar ]
- Poulin H., Bruhova I., Timour Q., Theriault O., Beaulieu J. M., Frassati D., et al. (2014). Fluoxetine blocks Nav1.5 channels via a mechanism similar to that of class 1 antiarrhythmics. Mol. Pharmacol. 86 378–389. 10.1124/mol.114.093104 [ PMC free article ] [ PubMed ] [ CrossRef ] [ Google Scholar ]
- Poupon L., Lamoine S., Pereira V., Barriere D. A., Lolignier S., Giraudet F., et al. (2018). Targeting the TREK-1 potassium channel via riluzole to eliminate the neuropathic and depressive-like effects of oxaliplatin. Neuropharmacology 140 43–61. 10.1016/j.neuropharm.2018.07.026 [ PubMed ] [ CrossRef ] [ Google Scholar ]
- Rennolds J., Tower C., Musgrove L., Fan L., Maloney K., Clancy J. P., et al. (2008). Cystic fibrosis transmembrane conductance regulator trafficking is mediated by the COPI coat in epithelial cells. J. Biol. Chem. 283 833–839. 10.1074/jbc.M706504200 [ PubMed ] [ CrossRef ] [ Google Scholar ]
- Rodrigues N., Bennis K., Vivier D., Pereira V., Chatelain C. F., Chapuy E., et al. (2014). Synthesis and structure-activity relationship study of substituted caffeate esters as antinociceptive agents modulating the TREK-1 channel. Eur. J. Med. Chem. 75 391–402. 10.1016/j.ejmech.2014.01.049 [ PubMed ] [ CrossRef ] [ Google Scholar ]
- Royal P., Andres-Bilbe A., Avalos Prado P., Verkest C., Wdziekonski B., Schaub S., et al. (2019). Migraine-associated TRESK mutations increase neuronal excitability through alternative translation initiation and inhibition of TREK. Neuron 101 : e236 . 10.1016/j.neuron.2018.11.039 [ PubMed ] [ CrossRef ] [ Google Scholar ]
- Sandoz G., Tardy M. P., Thummler S., Feliciangeli S., Lazdunski M., Lesage F. (2008). Mtap2 is a constituent of the protein network that regulates twik-related K+ channel expression and trafficking. J. Neurosci. 28 8545–8552. 10.1523/JNEUROSCI.1962-08.2008 [ PMC free article ] [ PubMed ] [ CrossRef ] [ Google Scholar ]
- Sandoz G., Thummler S., Duprat F., Feliciangeli S., Vinh J., Escoubas P., et al. (2006). AKAP150, a switch to convert mechano-, pH- and arachidonic acid-sensitive TREK K(+) channels into open leak channels. EMBO J. 25 5864–5872. 10.1038/sj.emboj.7601437 [ PMC free article ] [ PubMed ] [ CrossRef ] [ Google Scholar ]
- Santos R., Ursu O., Gaulton A., Bento A. P., Donadi R. S., Bologa C. G., et al. (2017). A comprehensive map of molecular drug targets. Nat. Rev. Drug Discov. 16 19–34. 10.1038/nrd.2016.230 [ PMC free article ] [ PubMed ] [ CrossRef ] [ Google Scholar ]
- Sauter D. R., Sorensen C. E., Rapedius M., Bruggemann A., Novak I. (2016). pH-sensitive K(+) channel TREK-1 is a novel target in pancreatic cancer. Biochim. Biophys. Acta 1862 1994–2003. 10.1016/j.bbadis.2016.07.009 [ PubMed ] [ CrossRef ] [ Google Scholar ]
- Schewe M., Nematian-Ardestani E., Sun H., Musinszki M., Cordeiro S., Bucci G., et al. (2016). A Non-canonical Voltage-Sensing Mechanism Controls Gating in K2P K(+) Channels. Cell 164 937–949. 10.1016/j.cell.2016.02.002 [ PMC free article ] [ PubMed ] [ CrossRef ] [ Google Scholar ]
- Shi D. N., Yuan Y. T., Ye D., Kang L. M., Wen J., Chen H. P. (2018). MiR-183-5p alleviates chronic constriction injury-induced neuropathic pain through inhibition of TREK-1. Neurochem. Res. 43 1143–1149. 10.1007/s11064-018-2529-4 [ PubMed ] [ CrossRef ] [ Google Scholar ]
- Song J. H., Huang C. S., Nagata K., Yeh J. Z., Narahashi T. (1997). Differential action of riluzole on tetrodotoxin-sensitive and tetrodotoxin-resistant sodium channels. J. Pharmacol. Exp. Ther. 282 707–714. [ PubMed ] [ Google Scholar ]
- Spillane J., Kullmann D. M., Hanna M. G. (2016). Genetic neurological channelopathies: molecular genetics and clinical phenotypes. J. Neurol. Neurosurg. Psychiatry 87 37–48. 10.1136/jnnp-2015-311233 [ PMC free article ] [ PubMed ] [ CrossRef ] [ Google Scholar ]
- Takahira M., Sakurai M., Sakurada N., Sugiyama K. (2005). Fenamates and diltiazem modulate lipid-sensitive mechano-gated 2P domain K(+) channels. Pflugers Arch. 451 474–478. 10.1007/s00424-005-1492-5 [ PubMed ] [ CrossRef ] [ Google Scholar ]
- Talley E. M., Solorzano G., Lei Q., Kim D., Bayliss D. A. (2001). Cns distribution of members of the two-pore-domain (KCNK) potassium channel family. J. Neurosci. 21 7491–7505. 10.1523/JNEUROSCI.21-19-07491.2001 [ PMC free article ] [ PubMed ] [ CrossRef ] [ Google Scholar ]
- Terrenoire C., Lauritzen I., Lesage F., Romey G., Lazdunski M. (2001). A TREK-1-like potassium channel in atrial cells inhibited by beta-adrenergic stimulation and activated by volatile anesthetics. Circ. Res. 89 336–342. 10.1161/hh1601.094979 [ PubMed ] [ CrossRef ] [ Google Scholar ]
- Tertyshnikova S., Knox R. J., Plym M. J., Thalody G., Griffin C., Neelands T., et al. (2005). BL-1249 [(5,6,7,8-tetrahydro-naphthalen-1-yl)-[2-(1H-tetrazol-5-yl)-phenyl]-amine]: a putative potassium channel opener with bladder-relaxant properties. J. Pharmacol. Exp. Ther. 313 250–259. 10.1124/jpet.104.078592 [ PubMed ] [ CrossRef ] [ Google Scholar ]
- Thummler S., Duprat F., Lazdunski M. (2007). Antipsychotics inhibit TREK but not TRAAK channels. Biochem. Biophys. Res. Commun. 354 284–289. 10.1016/j.bbrc.2006.12.199 [ PubMed ] [ CrossRef ] [ Google Scholar ]
- Tong L., Cai M., Huang Y., Zhang H., Su B., Li Z., et al. (2014). Activation of K(2)P channel-TREK1 mediates the neuroprotection induced by sevoflurane preconditioning. Br. J. Anaesth. 113 157–167. 10.1093/bja/aet338 [ PMC free article ] [ PubMed ] [ CrossRef ] [ Google Scholar ]
- Unudurthi S. D., Wu X., Qian L., Amari F., Onal B., Li N., et al. (2016). Two-Pore K+ Channel TREK-1 regulates sinoatrial node membrane excitability. J. Am. Heart Assoc. 5 : e002865 . 10.1161/JAHA.115.002865 [ PMC free article ] [ PubMed ] [ CrossRef ] [ Google Scholar ]
- Veale E. L., Al-Moubarak E., Bajaria N., Omoto K., Cao L., Tucker S. J., et al. (2014). Influence of the N terminus on the biophysical properties and pharmacology of TREK1 potassium channels. Mol. Pharmacol. 85 671–681. 10.1124/mol.113.091199 [ PubMed ] [ CrossRef ] [ Google Scholar ]
- Veyssiere J., Moha Ou Maati H., Mazella J., Gaudriault G., Moreno S., Heurteaux C., et al. (2015). Retroinverso analogs of spadin display increased antidepressant effects. Psychopharmacology 232 561–574. 10.1007/s00213-014-3683-2 [ PMC free article ] [ PubMed ] [ CrossRef ] [ Google Scholar ]
- Viatchenko-Karpinski V., Ling J., Gu J. G. (2018). Characterization of temperature-sensitive leak K(+) currents and expression of TRAAK, TREK-1, and TREK2 channels in dorsal root ganglion neurons of rats. Mol. Brain 11 : 40 . 10.1186/s13041-018-0384-5 [ PMC free article ] [ PubMed ] [ CrossRef ] [ Google Scholar ]
- Vierra N. C., Dadi P. K., Jeong I., Dickerson M., Powell D. R., Jacobson D. A. (2015). Type 2 diabetes–associated K + channel TALK-1 modulates β-cell electrical excitability, second-phase insulin secretion, and glucose homeostasis. Diabetes 64 3818–3828. 10.2337/db15-0280 [ PMC free article ] [ PubMed ] [ CrossRef ] [ Google Scholar ]
- Vivier D., Soussia I. B., Rodrigues N., Lolignier S., Devilliers M., Chatelain F. C., et al. (2017). Development of the first two-pore domain potassium channel TWIK-related K(+) channel 1-selective agonist possessing in vivo antinociceptive activity. J. Med. Chem. 60 1076–1088. 10.1021/acs.jmedchem.6b01285 [ PubMed ] [ CrossRef ] [ Google Scholar ]
- Wang M., Song J., Xiao W., Yang L., Yuan J., Wang W., et al. (2012). Changes in lipid-sensitive two-pore domain potassium channel TREK-1 expression and its involvement in astrogliosis following cerebral ischemia in rats. J. Mol. Neurosci. 46 384–392. 10.1007/s12031-011-9598-z [ PubMed ] [ CrossRef ] [ Google Scholar ]
- Wang W., Liu D., Xiao Q., Cai J., Feng N., Xu S., et al. (2018). Lig4-4 selectively inhibits TREK-1 and plays potent neuroprotective roles in vitro and in rat MCAO model. Neurosci. Lett. 671 93–98. 10.1016/j.neulet.2018.02.015 [ PubMed ] [ CrossRef ] [ Google Scholar ]
- Wang W., Zhang M., Li P., Yuan H., Feng N., Peng Y., et al. (2013). An increased TREK-1-like potassium current in ventricular myocytes during rat cardiac hypertrophy. J. Cardiovasc. Pharmacol. 61 302–310. 10.1097/FJC.0b013e318280c5a9 [ PubMed ] [ CrossRef ] [ Google Scholar ]
- Weckhuysen S., Mandelstam S., Suls A., Audenaert D., Deconinck T., Claes L. R., et al. (2012). KCNQ2 encephalopathy: emerging phenotype of a neonatal epileptic encephalopathy. Ann. Neurol. 71 15–25. 10.1002/ana.22644 [ PubMed ] [ CrossRef ] [ Google Scholar ]
- Wong D. T., Perry K. W., Bymaster F. P. (2005). Case history: the discovery of fluoxetine hydrochloride (Prozac). Nat. Rev. Drug Discov. 4 764–774. 10.1038/nrd1821 [ PubMed ] [ CrossRef ] [ Google Scholar ]
- Woo D. H., Han K. S., Shim J. W., Yoon B. E., Kim E., Bae J. Y., et al. (2012). TREK-1 and Best1 channels mediate fast and slow glutamate release in astrocytes upon GPCR activation. Cell 151 25–40. 10.1016/j.cell.2012.09.005 [ PubMed ] [ CrossRef ] [ Google Scholar ]
- Wu X., Liu Y., Chen X., Sun Q., Tang R., Wang W., et al. (2013). Involvement of TREK-1 activity in astrocyte function and neuroprotection under simulated ischemia conditions. J. Mol. Neurosci. 49 499–506. 10.1007/s12031-012-9875-5 [ PubMed ] [ CrossRef ] [ Google Scholar ]
- Xian Tao L., Dyachenko V., Zuzarte M., Putzke C., Preisig-Muller R., Isenberg G., et al. (2006). The stretch-activated potassium channel TREK-1 in rat cardiac ventricular muscle. Cardiovasc. Res. 69 86–97. 10.1016/j.cardiores.2005.08.018 [ PubMed ] [ CrossRef ] [ Google Scholar ]
- Xu X., Pan Y., Wang X. (2004). Alterations in the expression of lipid and mechano-gated two-pore domain potassium channel genes in rat brain following chronic cerebral ischemia. Brain Res. Mol. Brain Res. 120 205–209. 10.1016/j.molbrainres.2003.09.020 [ PubMed ] [ CrossRef ] [ Google Scholar ]
- Ye D., Li Y., Zhang X., Guo F., Geng L., Zhang Q., et al. (2015). TREK1 channel blockade induces an antidepressant-like response synergizing with 5-HT1A receptor signaling. Eur. Neuropsychopharmacol. 25 2426–2436. 10.1016/j.euroneuro.2015.09.007 [ PubMed ] [ CrossRef ] [ Google Scholar ]
- Zhou M., Xu G., Xie M., Zhang X., Schools G. P., Ma L., et al. (2009). TWIK-1 and TREK-1 are potassium channels contributing significantly to astrocyte passive conductance in rat hippocampal slices. J. Neurosci. 29 8551–8564. 10.1523/JNEUROSCI.5784-08.2009 [ PMC free article ] [ PubMed ] [ CrossRef ] [ Google Scholar ]
- Skip to main content
- Skip to header right navigation
- Skip to site footer
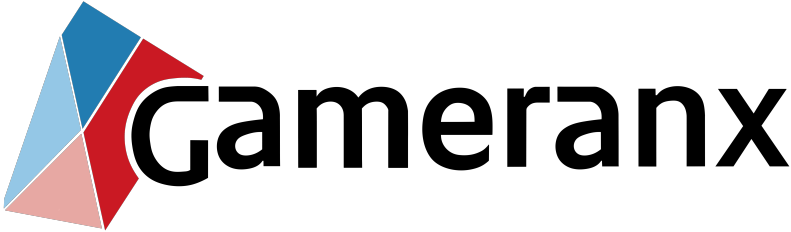
Video Game News, Lists & Guides
Trek to Yomi: All Chapter 2 Health and Stamina Upgrades
Improving Health and Stamina is important!
All Chapter 2 Health And Stamina Upgrades In Trek To Yomi
Stamina upgrade #1, stamina upgrade #2, stamina upgrade #3, health upgrade #1, stamina upgrade #4, health upgrade #2.
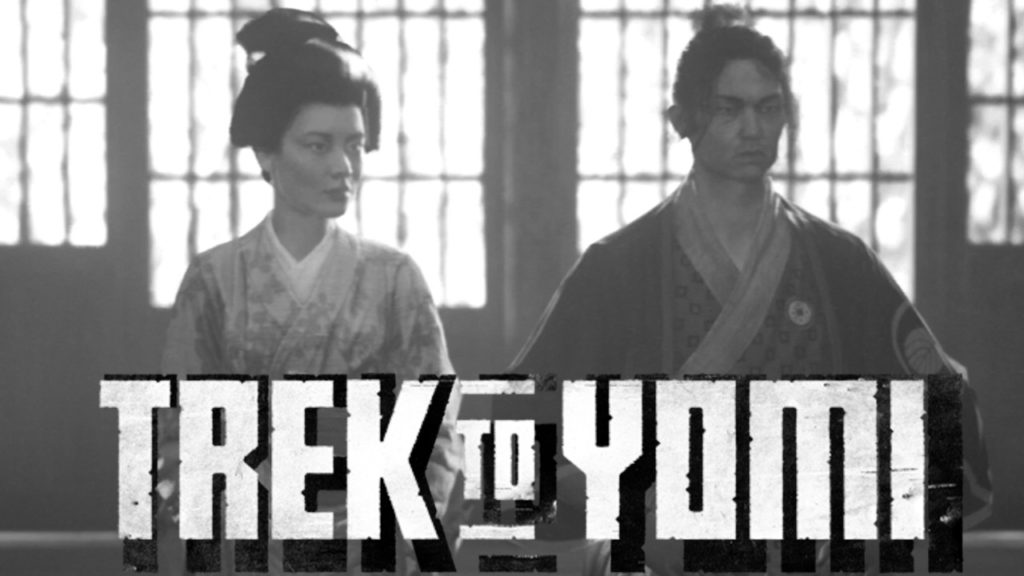
Out of all the collectibles that you can find in Trek to Yomi , arguably the most important are the Upgrades that will increase your Health and Stamina meters. Without constantly improving your stats throughout your journey, a first-time playthrough will see you getting cut down many times. There are a total of 33 Upgrades, with 22 of them being for your Stamina and the other 11 being for Health. Collecting all of these Upgrades will not only make you as powerful as you can be but also unlock the “Dedicated Collector” for collecting all of the game’s Upgrades.
In Chapter 2 of Trek to Yomi , Hiroki is sent to aid a neighboring village that is under siege. With his backup taken hostage, he will have to fight the enemy alone, making these Upgrades essential to his and your survival. This guide will show players where to find all of the possible Health and Stamina Upgrades found in Chapter 2 of Trek to Yomi .
There are a total of 6 Upgrades that you can find in the second chapter of Trek to Yomi . There are 4 Stamina Upgrades dotted around the Kamikawamura village and its surrounding forest, with the other 2 Upgrades being for your Health. Since you will not be able to revisit levels or zones once you go past them, this guide will show you where to find each Upgrade in the order that they appear in the level.
The first Stamina Upgrade is found immediately after getting control of Hiroki. After surviving the bandit ambush, go to the left to reach the screen pictured below. Sitting on a log in the middle of the screen is the first Stamina Upgrade for the chapter.
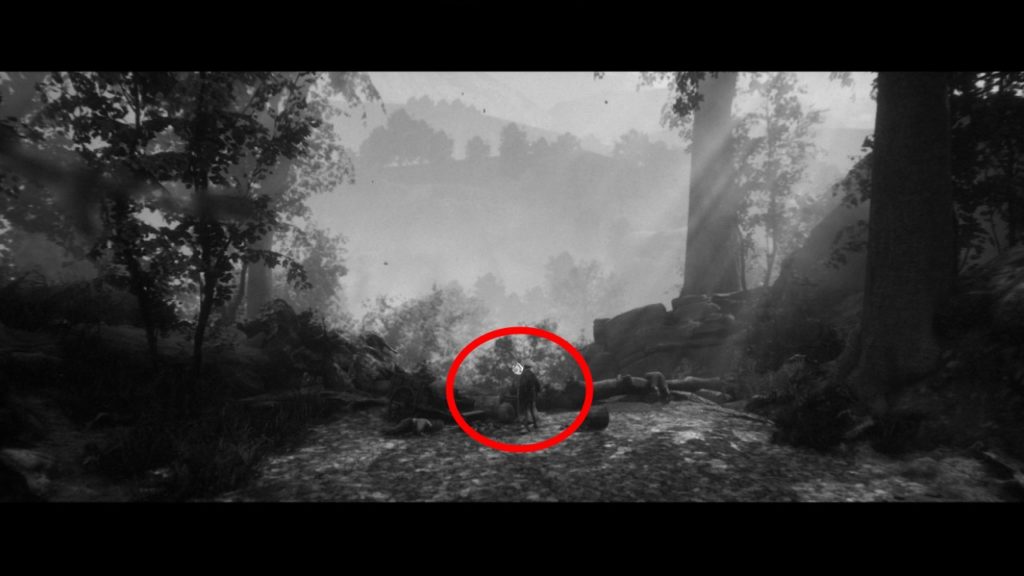
Continue through the chapter until you enter the mining tunnel found in the mountain. There is a group of bandits that have a prisoner tied up. Just beyond the enemies and civilian is an elevator that brings you up to a higher platform. Go to the right to leave the cave, the Stamina Upgrade will be directly in front of you once you exit.
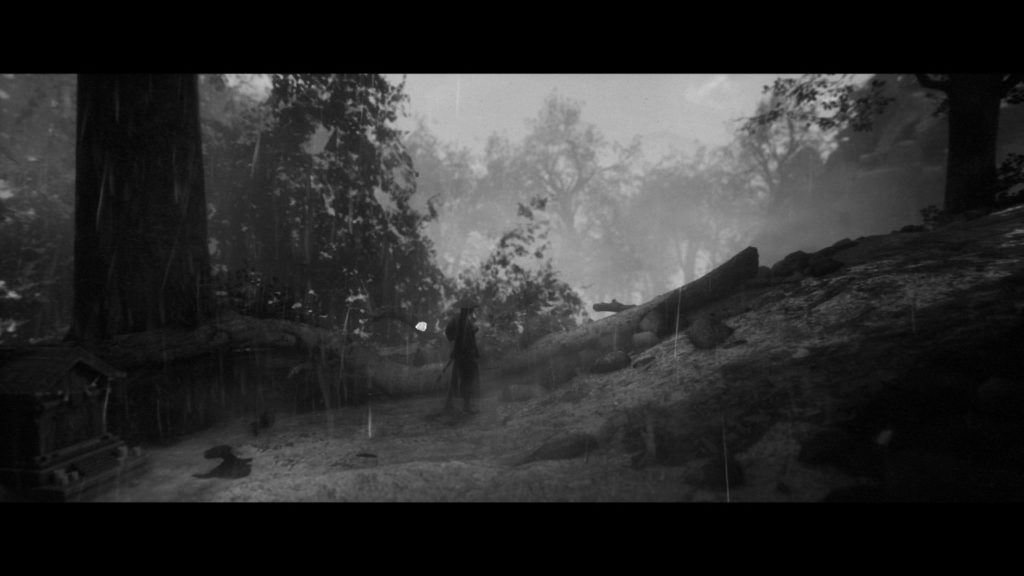
Continue through the level until you enter a cutscene where Hiroki sees his fellow samurai being taken into the village that you were sent to help. After the cutscene, make your way into the burning village. Upon entering the front gates of the village, go into the first house that you see, it is on top of a small hill. Inside you will find the Stamina Upgrade on a table.
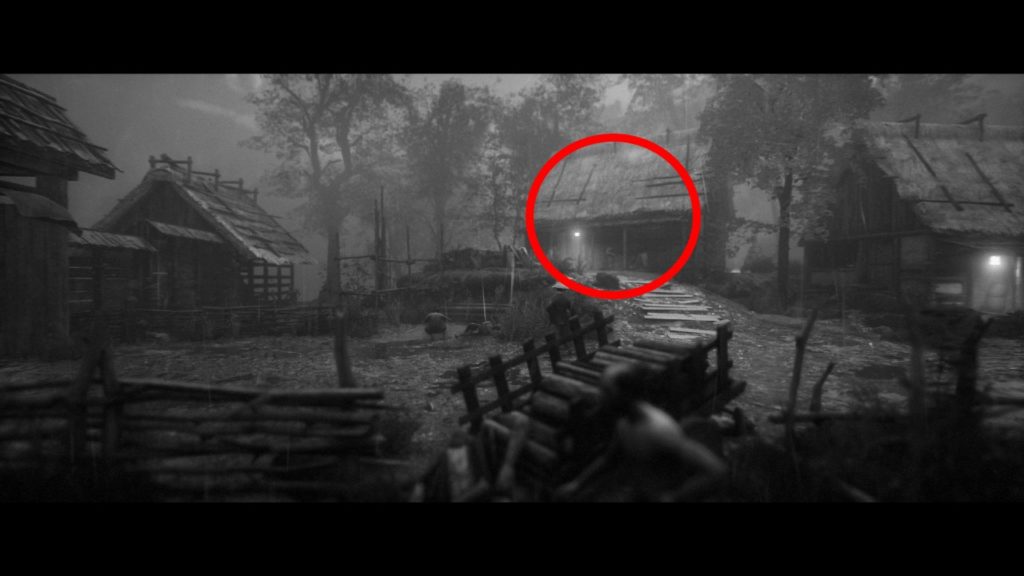
After fighting through the burning buildings that you fell through the roof of, you will come out to the opening in the first picture below. Go to the closed door on the left and kick it open. Run past the house and down the nearby dock. This is where you will find the chapter’s first Health Upgrade.
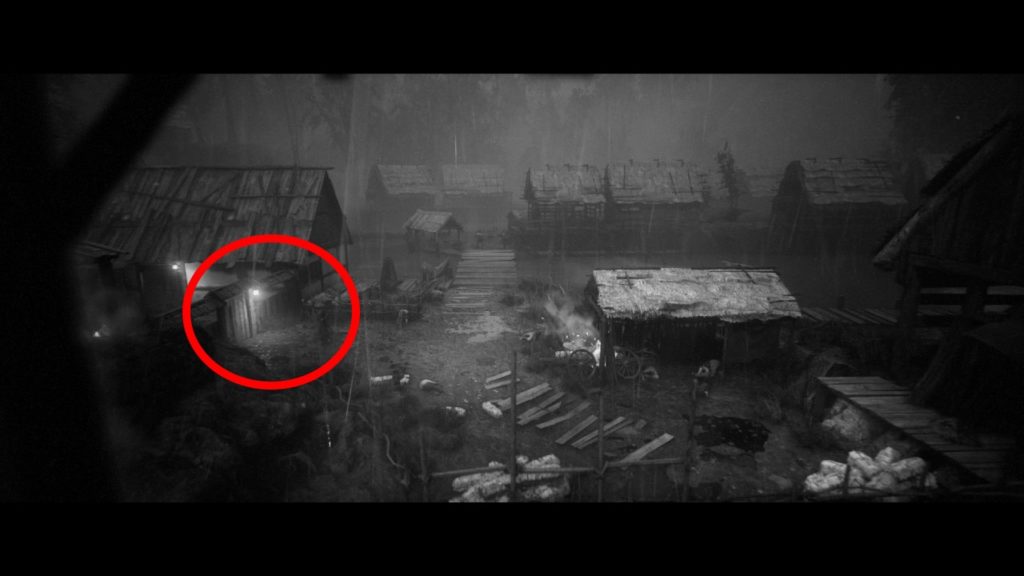
Continue through the village until you reach a bridge made out of boats. Before crossing the bridge that is going right, take the path on the left to reach the group of houses shown in the first picture down below. Enter the house on the left to find a dying man. On the table to his left is the last Stamina Upgrade for Chapter 2. This is also the location where you can find the Lost Love Letter Artifact.
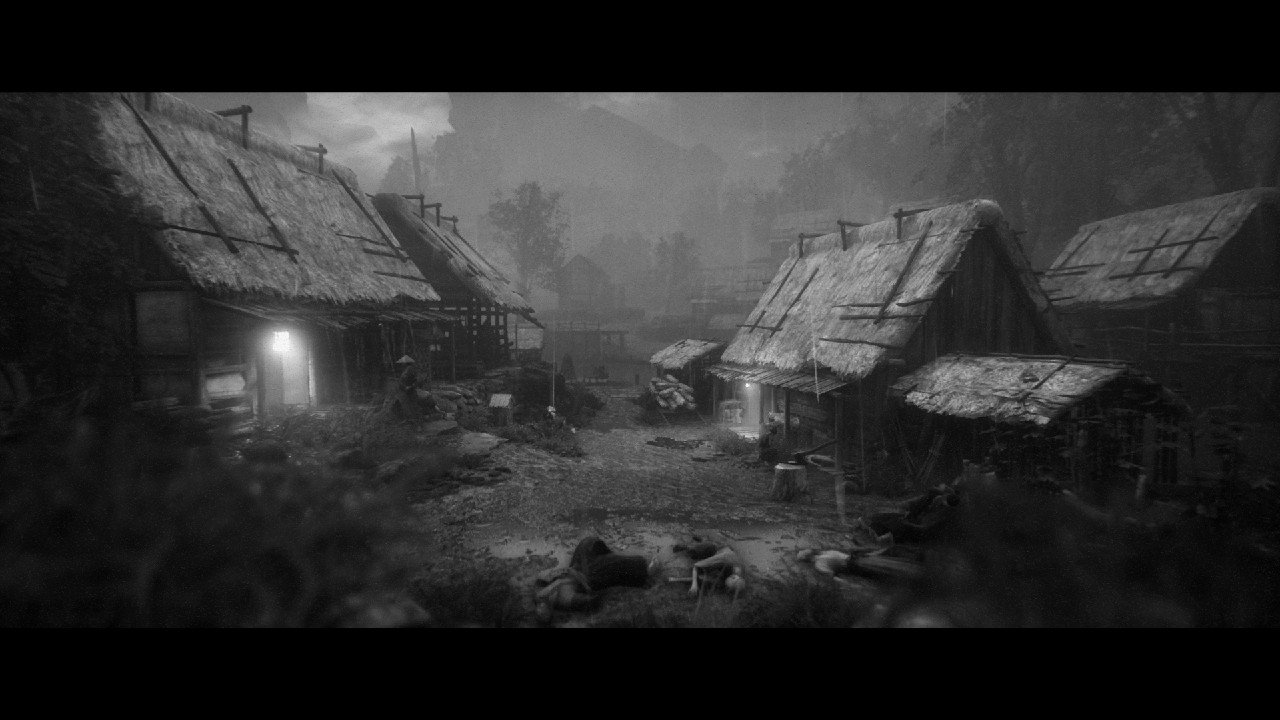
After picking up your first Fury Collectible that gives you the Double-side Block and Parry skill, you will run out of a barn and be directed to run up onto the rooftops. At the end of the roofs, you will cross a wooden bridge to reach a cliffside that has a flight of stairs leading up and leads to yet another wooden bridge. Just before the second bridge is a small crevice that you can squeeze through to find a number of items, including a shrine, an upgrade for your Bo-Shuriken, and the last Health Upgrade in the level.
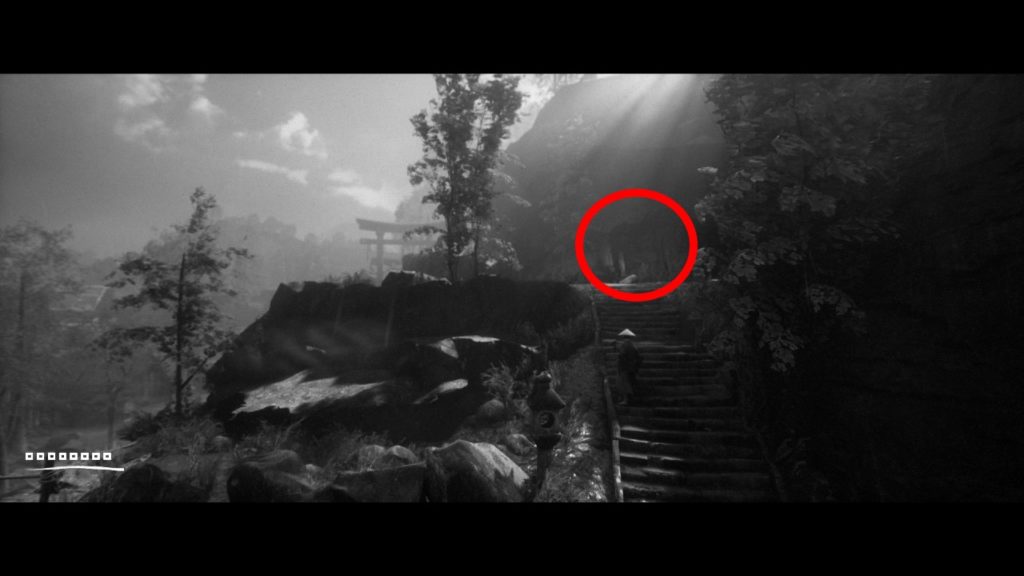
More Trek to Yomi guides:
Trek to Yomi: All Chapter 1 Health and Stamina Upgrades | Trek to Yomi: All Artifacts in Chapter 1 | Lore Collectibles Guide | Trek to Yomi: Full Achievement and Trophy List | Trek to Yomi: How to Get All 4 Endings | All Endings Explained | Trek to Yomi: All Artifacts in Chapter 2 | Lore Collectibles Guide
About Matt Villei
Matt Villei is a Guide Writer for Gameranx. Having a deep appreciation for the games, films, and books that he experienced growing up, Matt wanted to learn the inner workings of how these pieces of media were made. He graduated from Hofstra University with a Film Studies degree and a Creative Writing minor with the hope of one day making a game like the ones he grew up playing. When not writing, he plays some games, watches some professional wrestling, and spends some time with his two Golden Retrievers.

- Email Us

- Channels ►
- Executive Moves
- Transaction & Valuation
- Health Equity
- Patient Experience
- Care Coordination
- Legal & Regulatory
- Compensation
- Specialties ►
- Orthopedics
- Surgery Centers
- Dental / DSO
- Becker's Healthcare Websites ►
- Dental + DSO
- Behavioral Health
- Physician Leadership
- Newsletters ►
- Sign Up For Our Free E-Newsletters
- Hospital Review
- Hospital CEO Report
- Hospital CFO Report
- Health IT & CIO Report
- Clinical Leadership
- Revenue Cycle Management
- Digital Innovation Report
- Supply Chain
- Payer Issues
- Pharmacy Report
- Women's Leadership
- Laboratory Review
- Cardiology Report
- Oncology Report
- HR + Talent Review
- Post Acute Report
- Life Sciences Report
- Behavioral Health Report
- Marketing Report
- Events ►
- Upcoming Conferences and Events
- 14th Annual Meeting
- Spring Payer Issues Roundtable
- 21st Annual Spine, Orthopedic and Pain Management-Driven ASC + The Future of Spine Conference
- Spring Future of Dentistry Roundtable
- 9th Annual Health IT + Digital Health + RCM Annual Meeting: The Future of Business and Clinical Technologies
- Becker's ASC 30th Annual Meeting: The Business and Operations of ASCs
- Fall Future of Dentistry Roundtable
- Fall Payer Issues Roundtable
- 12th Annual CEO + CFO Roundtable
- Exhibiting & Sponsoring
- Call for Speakers
- Virtual Events ►
- Upcoming Virtual Events
- Digital Health + Telehealth Virtual Event
- Human Resources + Talent Virtual Event
- 14th Annual Meeting Virtual Event
- Payer Issues Virtual Event
- CMO + CNO Virtual Event
- CEO + CFO Virtual Forum
- Oncology Virtual Forum
- AI + Digital Health Virtual Event
- Digital Innovation + Patient Experience and Marketing Virtual Event
- Dental + DSO Virtual Event
- Past Virtual Events
- Webinars ►
- Upcoming Webinars
- OnDemand Webinars
- Partner Content ►
- Current Partner Content
- Podcasts ►
- Our Podcasts
- Becker's Healthcare Podcast Episodes
- Becker’s Digital Health + Health IT Podcast
- Becker’s Payer Issues Podcast
- Podcast Summaries
- Becker's Behavioral Health Podcast
- Becker's Ambulatory Surgery Centers Podcast
- Becker's Spine and Orthopedics Podcast
- Becker's Dental + DSO Review Podcast
- Becker’s Clinical Leadership Podcast
- Becker’s Pediatric Leadership Podcast
- Becker's Cardiology + Heart Surgery Podcast
- Becker's Women's Leadership Podcast
- Lists ►
- Nominations
- Sign up for list nomination updates
- Print ►
- Current Issue
- Past Issues
- Current Issue - Becker's Clinical Leadership
- Past Issues - Becker's Clinical Leadership
- Multimedia ►
- Intuitive + Becker's Content Hub
- NRC Health Content Hub
- Now is the Time
- LeanTaaS AI Solutions
- Healthcare Upside/Down Podcast Series
- Featured Content
- Career Center
- Mass General Brigham
- AMN Healthcare
- About Us ►
- About Becker's Hospital Review
- Careers at Becker's
- Request Media Kit
- Content Specifications
- Most Read ►
- 29 physician specialties ranked by annual compensation
- CMS pitches inpatient payment rule for 2025: 8 things to know
- Memorial Hermann says physician altered patient records, closed 2 transplant programs as a result
- Judy Faulkner's financial philosophy
- Why HCA nurses are working from home
- Uptick in mysterious condition puzzles physicians
- The 8 a.m. meeting debate: 5 health systems weigh in
- SSM Health market president heads to Dignity Health
- 'Fails to meet the moment': Hospital groups react to proposed inpatient payment rule
- Epic approved for 'otherworldly' expansion
- Top 40 Articles ►
- 6 hospitals seeking CEOs
- The best hospital in each state, per Newsweek
- Catholic health system to replace all crucifixes
- 8 drugs now in shortage
- California system gave $100,000 bonuses to nurses for retention. Did it work?
- UnitedHealth suspects 'nation-state' behind Change outage: 7 things to know
- 22 recent hospital, health system executive moves
- Jury ups Johns Hopkins hospital damages to $261M in Netflix case
- Experts scramble to understand rising cancer rates in young adults
- 22 hospitals, health systems cutting jobs
- Jury rules against Johns Hopkins in case made famous by Netflix
- Meet the 24-year-old running a Colorado hospital
- Former Kaiser nurse awarded $41M in retaliation lawsuit
- 15 'overpaid' CEOs in healthcare
- Mark Cuban: CEOs 'waste a sh-tload of money' on healthcare
- Walmart names 1st health system partner
- 52 hospitals with the most ED visits in 2022
- 9 women making moves in healthcare
- From -6.8% to 12.2%: 43 health systems ranked by operating margins
- Amazon launches One Medical for Prime
- 5 hospitals seeking CEOs
- 12 healthcare trends and issues we are following for 2024
- As Steward's finances stumble, spotlight turns to CEO's yachts
- 9 recent hospital, health system CEO moves
- 150+ top places to work in healthcare | 2024
- 'An attack on the entire sector': Fallout from Change Healthcare hack continues
- 100 largest hospitals and health systems in the US | 2023
- Best healthcare jobs in 2024: US News
- Healthgrades' 50 top hospitals for 2024
- UChicago sued for negligence in death of Silver Cross Hospital CEO
- 418 rural hospitals at risk of closure, breakdown by state
- 15 health systems with strong finances
- Ransomware group leader told hackers to attack hospitals, FBI, HHS say
- Where are Leapfrog's 18 straight-'A' hospitals?
- 146 hospitals, systems ranked by nurse satisfaction
- The No. 1 problem still keeping hospital CEOs up at night
- HHS intervenes in Change Healthcare hack
- The layoff runway lengthens
- Mark Cuban's drug company partners with 1st health system
- COO of Tennessee hospital gives resignation days after CEO
- Cybersecurity
- Digital Health
Trek Health raises $2.7M in seed funding
Mental health payment processing company Trek Health has raised $2.7 million in seed funding.
Trek Health works to automate the payment workflow for mental healthcare providers. The Trek Health platform can integrate with existing EHR systems, according to a Sept. 9 Trek Health news release.
Lionheart Ventures led the seed funding round.
"Mental health providers choose their profession to help those in need — not to get bogged down on manual tasks that should be automated just to get paid. Trek Health is working to serve healthcare providers and patients by bringing forward innovative fintech solutions and an intelligent auto-coding engine to facilitate better connections between providers and insurers, elevating the patient experience along the way," Trek Health founder and CEO Dilpreet Sahota said.
Copyright © 2024 Becker's Healthcare. All Rights Reserved. Privacy Policy . Cookie Policy . Linking and Reprinting Policy .
Featured Learning Opportunities
- Whitepapers
- Health IT Whitepapers
- Process Improvement Whitepapers
- Finance Whitepapers
- Clinical Whitepapers
- Payer Whitepapers
- Other Learning Opportunities
- Health IT Webinars
- Process Improvement Webinars
- Finance Webinars
- Clinical Webinars
- Payer Webinars
- Virtual Events
- ASC/Spine Whitepapers
- Dental Whitepapers
- ASC/Spine Webinars
- Dental Webinars
- Hospital Review Virtual Events
- ASC/Spine Virtual Events
- Dental Virtual Events
Featured Whitepapers
Featured webinars.
Becker's Websites
Virtual Learning
Conferences
- 9th Annual Health IT + Digital Health + RCM Meeting: The Future of Business and Clinical Technologies
- 1.800.417.2035
- [email protected]

Trek Health’s platform integrates with EHR systems and provides a suite of solutions for mental health providers including instant claim reimbursement, automatic claim generation and submissions, and payer enrollments across 900+ insurance networks. The company has seen an early surge in demand, serving a broad set of customers across the United States including inpatient mental health rehabilitation centers, outpatient psychiatric facilities, outpatient psychotherapy facilities, and venture capital-backed mental health startups.
ABOUT TREK HEALTH
Trek Health is delivering a frictionless financial experience between mental health providers, patients and payers. The company eliminates administrative burdens required for financial transactions to ensure providers are able to maximize their time with patients. By leveraging artificial intelligence to automate claims processing, Trek Health is synchronizing providers and payers to ensure the highest quality of mental health care is delivered nationwide. Connect with Trek Health at trekhealth.io !
Dilpreet Sahota, CEO & Founder [email protected]
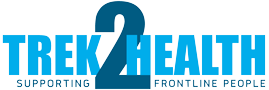
- Our Expertise
- Our Ambassadors
- Our Sponsors
- Our Partners
- Our Services
- Partner with us
- 6 Week Frontline Program
- QPS Day Program
- Plumbing Day Program
- 6 Week Plumbing Program
- Malibu Raffle now open!
- Midnight to Dawn ANZAC Service Trek 2024
- Aussie Eco Surfboards – Trek Shed
- Parking info for Enoggera Reservoir Treks
- International edition
- Australia edition
- Europe edition
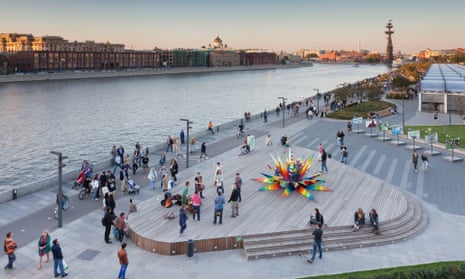
'Things have started to improve': Moscow residents share thoughts on the city's changes
From cycling infrastructure to public spaces, how do Moscow’s residents feel about the city’s urban renewal projects? Here they share their stories
The ‘Moscow experiment’ has seen the city undergo renewal efforts over the last five years to improve liveability. But what do Moscow’s residents really think about the changes? We asked you to share your stories of life in the Russian capital, and reflect on whether Moscow is changing for better or worse.
The city centre may be seeing improvements in infrastructure and public space, but what about the suburbs? How involved have local citizens been in the changes? And what does the future hold for Moscow? We’ve rounded up a selection of your GuardianWitness contributions , comments and emails, which reveal the everyday experiences of Moscow’s transformations:
About pedestrians and automobilists
Moscow has undergone massive change in the last five years, but the most obvious developments concern parks, streets, and general navigation in the capital. Most of the developments are surely positive: the city has seemed to get more air. There have appeared more walking paths, pedestrian zones have been expanded, some streets have been closed for traffic altogether, parks are being renovated, and new bicycle lanes are being offered now to city residents and its guests. However, there are negative changes as well, which have largely affected car owners: extension of the paid parking areas, and the increase of the average price, the need to navigate a bypass route in order to drive round pedestrian zones. But this coin has another side too: fewer cars in the centre means less exhaust fumes and cleaner air. Muscovites look forward to the old parks being renovated, and the new ones being opened. New plans make excited everyone: new metro stations, new roads, new bicycle lanes and pedestrian footpaths.
It's changing for better
Moscow is definitely changing for better now. As I see it, the authorities are trying to make a city a better place to live in, especially in remote residential neighborhoods, which is very good. Many parks were renovated, cycle lanes appeared. Moscow has become a nice place for long walks and cycling. The city has a lot to offer now including museums, and different events like exhibitons, summer outside activities and others. It's a pity that it takes so long but taking into account the size of the city and its population I can say the situation has changed even if compared to what it was like 4-6 years ago.
I grew up in Moscow and lived here until two years ago. My general impression is that the people making the decisions are trying to copy some European practices like bike rental, parks, etc, but the general success is limited. The streets still belong to drivers, not to pedestrians, to an unbelievable extent. Another thing is that street retail was banned in an attempt to improve the city. It works for the city centre, where retailers really spoiled the overall impression of the scene, but 80% of Moscow is not a city centre. And there, this removal makes your life more difficult. The positive trend is all these multiple festivals, exhibitions, etc, but the best of all these events were initiated by citizens, so I would say that it is due to improving integration of Muscovites into the global world. Overall, I should admit that I love my homeland, but there is still a far way to go to make the city as comfortable as it could be, and the incentives of the people in charge in many cases do not have a lot to do with the wellbeing of Muscovites. - Ekaterina Ivanova
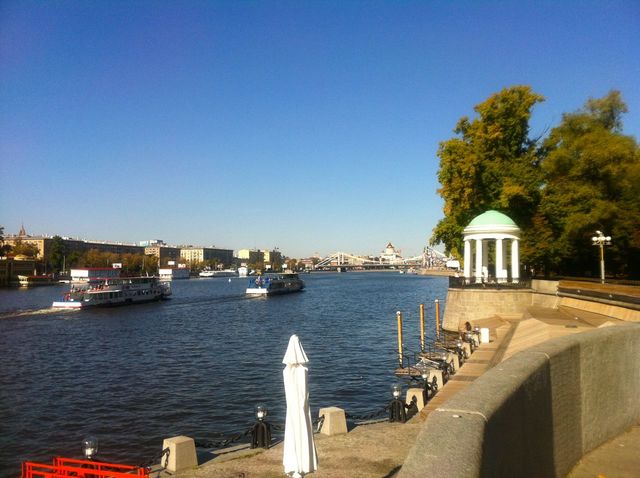
In the city
Gorky central park of culture and leisure
Better, definitely.
I was born in Moscow, emigrated to the UK ten years ago, and have been coming back at least once a year ever since. Although it's hard to tell from only a short visit, I can definitely see improvement in the capital: public transport operates better, local government services are better organised, the streets are cleaner... in my old neighbourhood (a very working-class, high-immigrant community), there are more 'high-street' shops appearing, less potholes on the roads, new playgrounds, new trees being planted and even the occasional fountain being built. These things may seem little and shallow, but I can certainly see improvement in this sense, at least.
It’s better on the surface, but still has inclusion and institutional issues. What do I mean? I mean that no one really asked us, Moscow residents, about the changes being made in the city (although we really welcome a big part of them). And lack of inclusion and mutual trust between the residents is in the end the main problem of Moscow as a city and a community (in my opinion). You can build as much fancy stuff as the money allows you to but if the Muscovites do not trust each other and don’t consider this city to truly belong to them, it will never become a real city and will continue to exist as “a big village” with fancy parks, craft beer and bicycle service. - Sergey Kurakov
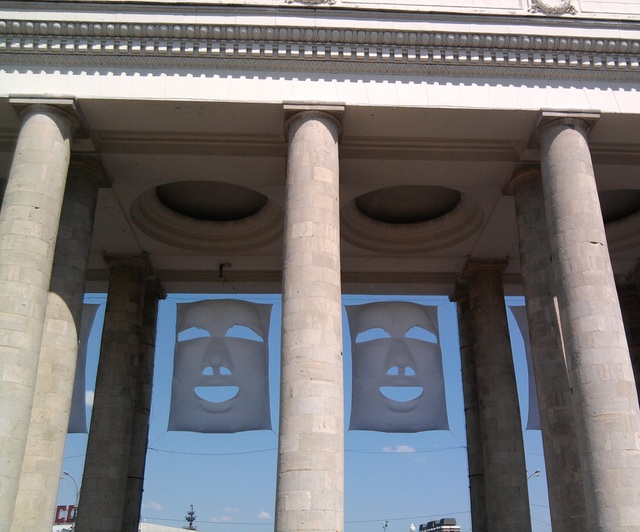
When you smiling
Moscow is beautiful with the history, but it's even more beautiful when history meets modern cultural programmes. This installation is a part of one of them. Such things can be seen in the city when we have holidays
The transformation of Gorky park was nice but it is now overly popular which means it is not so pleasant to go to. The boulevard ring has in the main been transformed and makes for a pleasant walk. The banks of the Moskva have been transformed and the walk from Red October down to Gorky park is now interesting, however I always feel that Moscow does not make as much as it's river as other cities (Paris or London for example) do. The area around modern tretyakov could be transformed into an area more like the south bank.
However the biggest killer of Moscow life are simply the size of the roads. They are everywhere and take priority over pedestrians. To really improve Moscow life would be deal with the massive structural change required for dealing with traffic. And that, unfortunately, costs more than plants.

I was born in Moscow and truly love it. And this city inspired me to take an urban planning course: I honestly just couldn’t figure out how one of the wealthiest cities in the whole world could experience such a plethora of problems ranging from car invasion to banal low visual and urban design culture. Many things have changed for the better. A lot of new creative things emerged, such as anti-cafes, high-quality bars and famous Moscow art-clusters. These all influence the city and make it somewhat more democratic. On top of that, there are more direct influences on its physical fabric due to new urban design studios like StrelkaKB, Wowhaus and many others. However, the overall system is still underdeveloped. Moscow is not really clean, it is over-reliant on cars and it seems not much action is taken to improve that; it’s incredibly unfriendly to wheelchairs and people of similar mobility. We still get enormous unnecessary shopping malls planted close to the centre. Moscow is a wonderful city and sure enough it is gradually developing, however, only in a discrete, tactical way, largely and mostly driven by private interests. The public sector, in turn, seems to provide no clear strategic policy to turn Moscow in a healthy modern democratic city with all its problems tackled in one way or another. - dmitryBooM
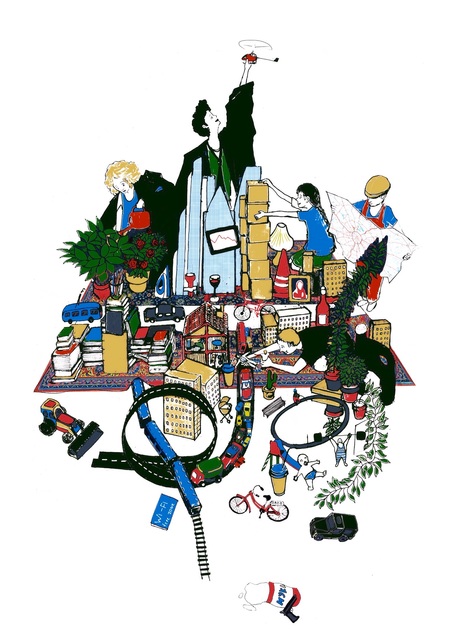
Comments (…)
Most viewed.
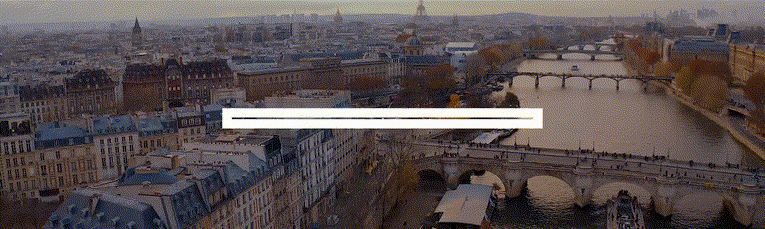
COMMENTS
Register now for Midnight to Dawn Anzac Service Trek 2024 Building Mental Resilience Your Path to a Healthier Mind Trek2Health, a Brisbane-based non-profit, has been leading the charge in improving the mental health and well-being of frontline workers and veteran communities for the last three years. Learn more Mental Focus Our Mission Client Services Donate…
Your Journey to Wellness. Our approach involves progressive training in a supportive, stigma-free environment. You'll undertake four modules, each lasting six weeks, with a walking challenge at the end of each. This progressive challenge keeps you motivated to improve your resilience and wellness over 12 months.
Trek2Health. Trek2Health. 407 likes · 14 talking about this · 44 were here. Providing stigma-free mental wellness support services for QLD veterans & frontline workers.
Optimize Healthcare Financials, Enhance Patient Care. Unify financial oversight and patient engagement with Trek Health. Our dual solutions in RCM and Payer Transparency streamline payment cycles and unlock market insights, enhancing revenue while you provide exceptional care. Insightful Data for Strategic Decisions.
Trek Health | 7,826 followers on LinkedIn. Smart payments solutions for healthcare. | Revolutionizing healthcare with two key products: Revenue Cycle Management (RCM) and Payer Transparency Analytics.
🎊 ANNOUNCING OUR MIDNIGHT TO DAWN ANZAC SERVICE TREK 🎊 Registrations are now open for our annual April 25th trek! This is a 20km night trek from Anzac Square to Mt Cootha, finishing at Anzac Memorial Park, The Gap. ... Trek2Health works to provide support for the health and well-being of the Emergency Services and Veteran communities in ...
Contact Us Frequently Asked Questions I am seeking information about Trek2Health services for Emergency services personnel. If you or someone you know would benefit from Trek2Health resilience programs, please get in touch. Our services are open to current and ex-serving emergency services personnel and veterans. Click here for more details about our services for Frontline…
For Providers. At Trek Healthcare, we provide patient management solutions that allow you to focus on high-quality, value-based care your patients will grow to love. Our goal is to streamline your primary care practice with the proven tools and support that elevate your capabilities, improve patient outcomes, and expand your practice.
Trek-1 Channel. TREK-1, named KCNK2 or K 2P 2.1 belongs to a large family of K 2P channels containing 15 members grouped in six subfamilies. K 2P channels are the most recent class of K + channels discovered. K 2P channels or the two-pore domain potassium channels are tandems of four transmembrane segments (M1-M4) containing two-pore domain (P1 and P2) (Figure 1, ,2). 2).
All Chapter 2 Health And Stamina Upgrades In Trek To Yomi. There are a total of 6 Upgrades that you can find in the second chapter of Trek to Yomi.There are 4 Stamina Upgrades dotted around the ...
Email. Mental health payment processing company Trek Health has raised $2.7 million in seed funding. Trek Health works to automate the payment workflow for mental healthcare providers. The Trek ...
Since the pandemic, which accelerated the shift to a virtual existence, people are moving less than ever, Gibbs said. Just 1 in 4 men and 1 in 5 women and adolescents currently get the recommended amount of aerobic and muscle-strengthening exercise, the federal guidelines say. "We have engineered physical activity out of our lives," Gibbs said.
SAN MATEO, Calif.--(BUSINESS WIRE)--Trek Health, the payment and insurance workflow automation platform for mental health provider groups, has closed an oversubscribed $2.7M Seed Round led by ...
2. BHP Group. View Full List. Top 10 companies in Australia by number of employees. 1. EY Australia. 2. Woolworths Group. View Full List. Top 10 companies in Australia by total funding amount. 1. Ramsay Health Care. 2. Roy Hill. View Full List. See top companies. Frequently Asked Questions regarding Trek2Health.
0401 713 039. For general enquiries email: [email protected]. For client enquiries email: [email protected]. Client Programs. 6 Week Frontline Program. 6 Week Plumbing Industry Program. QPS Day Program. Plumbing Day Program. Join Us.
Trek2Health Ltd IS ESTABLISED AS ON 31-Jul-2019 AS A charity IN Queensland and ABN NO OF Trek2Health Ltd IS 41635257046. Trek2Health's Client Program Report for 2023 highlights the organisation's commitment to improving mental health and resilience among front-line workers in Australia. Resilience, the ability to navigate life's challenges, is central to Trek2Health's philosophy. The ...
Our Health Trek challenges feature a variety of health- and safety-related ideas for children and adults. We'll release new challenges every 1-2 weeks, but you can work through them at your own pace, too. How to do the health trek. Throughout the year, complete a challenge and put the matching sticker on your gameboard. Challenges will help you ...
Walking tour around Moscow-City.Thanks for watching!MY GEAR THAT I USEMinimalist Handheld SetupiPhone 11 128GB https://amzn.to/3zfqbboMic for Street https://...
WHEREAS, under Moscow City Code section 2-I-7 and Idaho Code 50-304,the City may pass all ordinances and make all regulations necessary to preserve the public health; prevent the introduction of contagious diseases into the city; make quarantine laws for that purpose and enforce the same within five (5) miles of the city; and
There are lots to see in the city centre of Moscow, so we decided to start our series of Russia travel videos by showing you around the most historical part ...
For Providers. At Trek Healthcare, we provide patient management solutions that allow you to focus on high-quality, value-based care your patients will grow to love. Our goal is to streamline your primary care practice with the proven tools and support that elevate your capabilities, improve patient outcomes, and expand your practice.
Moscow has undergone massive change in the last five years, but the most obvious developments concern parks, streets, and general navigation in the capital.