How Light's Speed was Determined
Hsc physics syllabus.
Conduct investigations of historical and contemporary methods used to determine the speed of light and its current relationship to the measurement of time and distance. (ACSPH082)
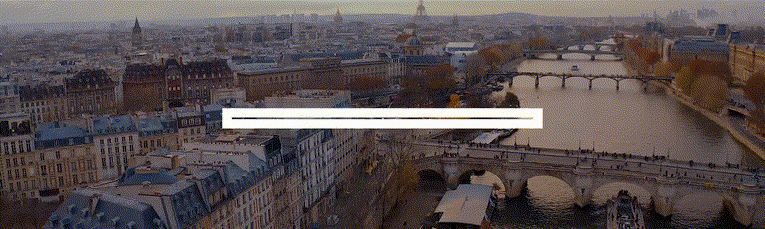
Experiments that Determined the Speed of Light
Historical Methods to Measure Light's Speed
1675 – ole rømer 200,000 km/sec.
Ole Rømer was a Danish astronomer who was observing the orbital motion of Io (one of the four satellites of Jupiter), when he determined the speed of light. The orbital period of Io around Jupiter is about 1.7 Earth days, and during each orbit, Io is eclipsed by Jupiter once as viewed from Earth.

Diagram source: Shea, J.H., 1998. Ole Rømer, the speed of light, the apparent period of Io, the Doppler effect, and the dynamics of Earth and Jupiter. American Journal of Physics 66, 561–569 .

Figure: Left: Longest apparent orbital period is observed when Earth's receding velocity (moving away) relative to Jupiter is the greatest. Right: Shortest apparent orbital period of Io is observed when Earth's relative velocity towards Jupiter is the greatest.
1728 – James Bradley 301,000 km/sec

Figure: Rest frame refers to the true position of a star while moving frame refers to the displaced perceived position of a star due to the relative velocity of Earth. The latter observation is called stellar aberration.
1849 – Hippolyte Louis Fizeau 313,300 km/sec
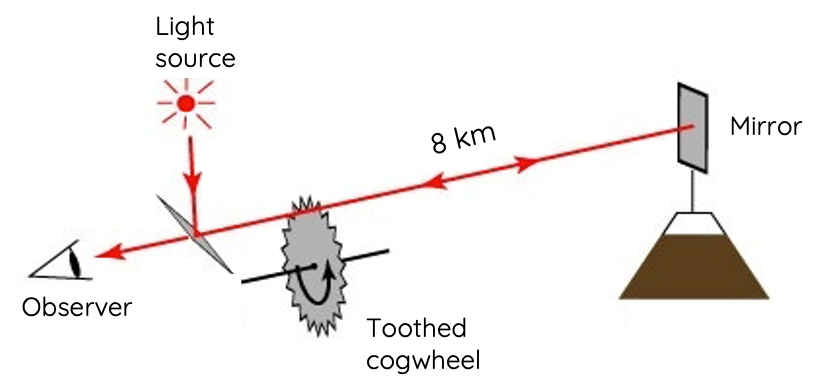
1862 – Leon Foucault 298,000 km/sec
Foucault’s experiment exploited the same principle as Fizeau, but he utilised rotating mirrors instead of a toothed wheel.

Contemporary Methods to Measure Light's Speed
1907 – rosa & dorsey 299,788 km/sec.
The scientific breakthrough in electromagnetism by James Maxwell in 1860s shed light on a new way of measuring speed of light. Rosa and Dorsey used the electric permittivity ( ε ) and magnetic permeability ( µ ) constants to calculate the speed of light.
$$c=\frac{1}{\sqrt{εμ}}$$
This method differed from historical methods in that it did not require an measurement of light's time of flight.
1958 – Froome 299,972,500 m/s
1973 – evenson et al 299,972,457 m/s.
Evenson et al utilised lasers with high spectral stability to measure the speed of light. Like Froome, this experiment also involved interferometry to measure the wavelength of light.

Figure: simplified experimental set-up for interferometry used to measure light's speed.
Light’s Relationship with Time and Distance
- In the modern age, due to the constancy of light’s speed, standard distance and time units are defined using speed of light.
- The concept of distance and time is not constant as they change with frames of reference. This is due to Albert Einstein's theory of Special Relativity and his postulate - speed of light is constant in a vacuum, regardless of the observer. This will be covered in more detail later in the module.
Constancy of light has two effects:
- Time becomes dilated (each second becomes longer) when an observer is at relativistic motion with the observed frame of reference.
- Length becomes shorter when an observer is travelling at relativistic motion.
As a result, distance (metre) and time (second) are measured and defined in terms of speed of light, which is a constant value.
- The definition of a metre is the distance light travels in 1/299,792,458 second.
- The definition of a second is the time light takes to travel 299,792,458 metres.
Previous section: Maxwell's Contribution to Electromagnetism
Next section: Introduction to Spectroscopy
- choosing a selection results in a full page refresh
- press the space key then arrow keys to make a selection
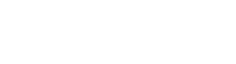
- Login/Register
- Solar System
- Exotic Objects
- Upcoming Events
- Deep-Sky Objects
- Observing Basics
- Telescopes and Equipment
- Astrophotography
- Space Exploration
- Human Spaceflight
- Robotic Spaceflight
- The Magazine
What is the speed of light? Here’s the history, discovery of the cosmic speed limit
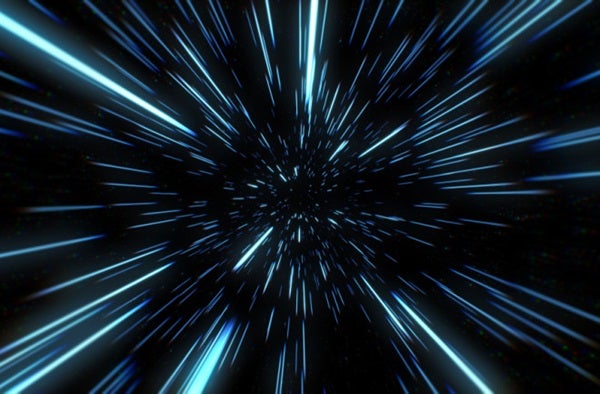
On one hand, the speed of light is just a number: 299,792,458 meters per second. And on the other, it’s one of the most important constants that appears in nature and defines the relationship of causality itself.
As far as we can measure, it is a constant. It is the same speed for every observer in the entire universe. This constancy was first established in the late 1800’s with the experiments of Albert Michelson and Edward Morley at Case Western Reserve University . They attempted to measure changes in the speed of light as the Earth orbited around the Sun. They found no such variation, and no experiment ever since then has either.
Observations of the cosmic microwave background, the light released when the universe was 380,000 years old, show that the speed of light hasn’t measurably changed in over 13.8 billion years.
In fact, we now define the speed of light to be a constant, with a precise speed of 299,792,458 meters per second. While it remains a remote possibility in deeply theoretical physics that light may not be a constant, for all known purposes it is a constant, so it’s better to just define it and move on with life.
How was the speed of light first measured?
In 1676 the Danish astronomer Ole Christensen Romer made the first quantitative measurement of how fast light travels. He carefully observed the orbit of Io, the innermost moon of Jupiter. As the Earth circles the Sun in its own orbit, sometimes it approaches Jupiter and sometimes it recedes away from it. When the Earth is approaching Jupiter, the path that light has to travel from Io is shorter than when the Earth is receding away from Jupiter. By carefully measuring the changes to Io’s orbital period, Romer calculated a speed of light of around 220,000 kilometers per second.
Observations continued to improve until by the 19 th century astronomers and physicists had developed the sophistication to get very close to the modern value. In 1865, James Clerk Maxwell made a remarkable discovery. He was investigating the properties of electricity and magnetism, which for decades had remained mysterious in unconnected laboratory experiments around the world. Maxwell found that electricity and magnetism were really two sides of the same coin, both manifestations of a single electromagnetic force.
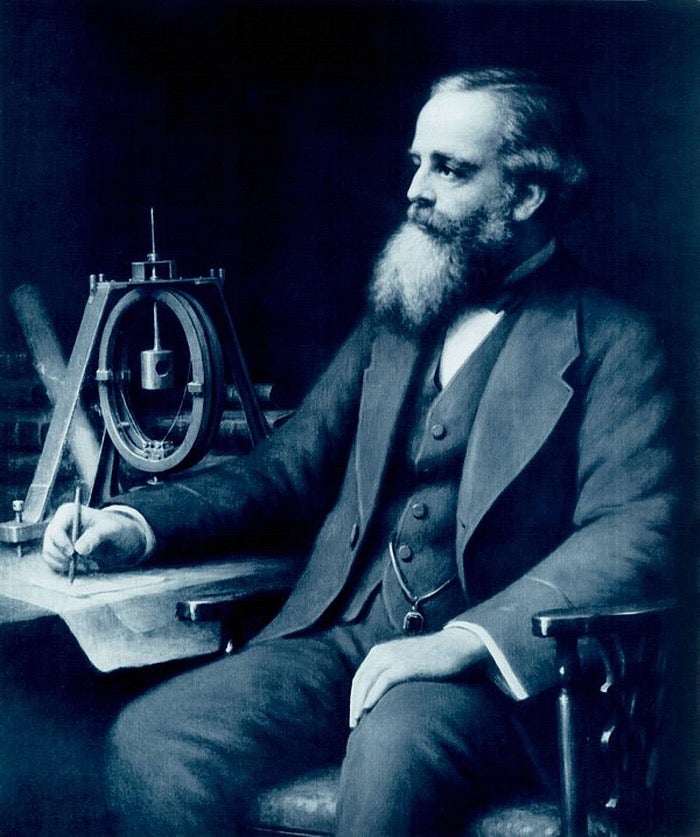
As Maxwell explored the consequences of his new theory, he found that changing magnetic fields can lead to changing electric fields, which then lead to a new round of changing magnetic fields. The fields leapfrog over each other and can even travel through empty space. When Maxwell went to calculate the speed of these electromagnetic waves, he was surprised to see the speed of light pop out – the first theoretical calculation of this important number.
What is the most precise measurement of the speed of light?
Because it is defined to be a constant, there’s no need to measure it further. The number we’ve defined is it, with no uncertainty, no error bars. It’s done. But the speed of light is just that – a speed. The number we choose to represent it depends on the units we use: kilometers versus miles, seconds versus hours, and so on. In fact, physicists commonly just set the speed of light to be 1 to make their calculations easier. So instead of trying to measure the speed light travels, physicists turn to more precisely measuring other units, like the length of the meter or the duration of the second. In other words, the defined value of the speed of light is used to establish the length of other units like the meter.
How does light slow down?
Yes, the speed of light is always a constant. But it slows down whenever it travels through a medium like air or water. How does this work? There are a few different ways to present an answer to this question, depending on whether you prefer a particle-like picture or a wave-like picture.
In a particle-like picture, light is made of tiny little bullets called photons. All those photons always travel at the speed of light, but as light passes through a medium those photons get all tangled up, bouncing around among all the molecules of the medium. This slows down the overall propagation of light, because it takes more time for the group of photons to make it through.
In a wave-like picture, light is made of electromagnetic waves. When these waves pass through a medium, they get all the charged particles in motion, which in turn generate new electromagnetic waves of their own. These interfere with the original light, forcing it to slow down as it passes through.
Either way, light always travels at the same speed, but matter can interfere with its travel, making it slow down.
Why is the speed of light important?
The speed of light is important because it’s about way more than, well, the speed of light. In the early 1900’s Einstein realized just how special this speed is. The old physics, dominated by the work of Isaac Newton, said that the universe had a fixed reference frame from which we could measure all motion. This is why Michelson and Morley went looking for changes in the speed, because it should change depending on our point of view. But their experiments showed that the speed was always constant, so what gives?
Einstein decided to take this experiment at face value. He assumed that the speed of light is a true, fundamental constant. No matter where you are, no matter how fast you’re moving, you’ll always see the same speed.
This is wild to think about. If you’re traveling at 99% the speed of light and turn on a flashlight, the beam will race ahead of you at…exactly the speed of light, no more, no less. If you’re coming from the opposite direction, you’ll still also measure the exact same speed.
This constancy forms the basis of Einstein’s special theory of relativity, which tells us that while all motion is relative – different observers won’t always agree on the length of measurements or the duration of events – some things are truly universal, like the speed of light.
Can you go faster than light speed?
Nope. Nothing can. Any particle with zero mass must travel at light speed. But anything with mass (which is most of the universe) cannot. The problem is relativity. The faster you go, the more energy you have. But we know from Einstein’s relativity that energy and mass are the same thing. So the more energy you have, the more mass you have, which makes it harder for you to go even faster. You can get as close as you want to the speed of light, but to actually crack that barrier takes an infinite amount of energy. So don’t even try.
How is the speed at which light travels related to causality?
If you think you can find a cheat to get around the limitations of light speed, then I need to tell you about its role in special relativity. You see, it’s not just about light. It just so happens that light travels at this special speed, and it was the first thing we discovered to travel at this speed. So it could have had another name. Indeed, a better name for this speed might be “the speed of time.”
Related: Is time travel possible? An astrophysicist explains
We live in a universe of causes and effects. All effects are preceded by a cause, and all causes lead to effects. The speed of light limits how quickly causes can lead to effects. Because it’s a maximum speed limit for any motion or interaction, in a given amount of time there’s a limit to what I can influence. If I want to tap you on the shoulder and you’re right next to me, I can do it right away. But if you’re on the other side of the planet, I have to travel there first. The motion of me traveling to you is limited by the speed of light, so that sets how quickly I can tap you on the shoulder – the speed light travels dictates how quickly a single cause can create an effect.
The ability to go faster than light would allow effects to happen before their causes. In essence, time travel into the past would be possible with faster-than-light travel. Since we view time as the unbroken chain of causes and effects going from the past to the future, breaking the speed of light would break causality, which would seriously undermine our sense of the forward motion of time.
Why does light travel at this speed?
No clue. It appears to us as a fundamental constant of nature. We have no theory of physics that explains its existence or why it has the value that it does. We hope that a future understanding of nature will provide this explanation, but right now all investigations are purely theoretical. For now, we just have to take it as a given.
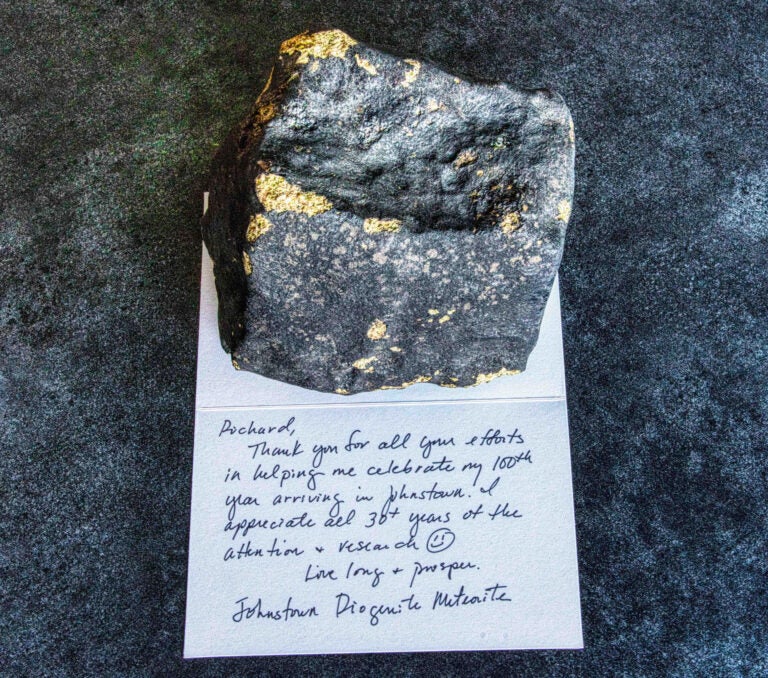
Colorado town throws 100th birthday party for pet space rock

The legacy of the Advanced Imaging Conference
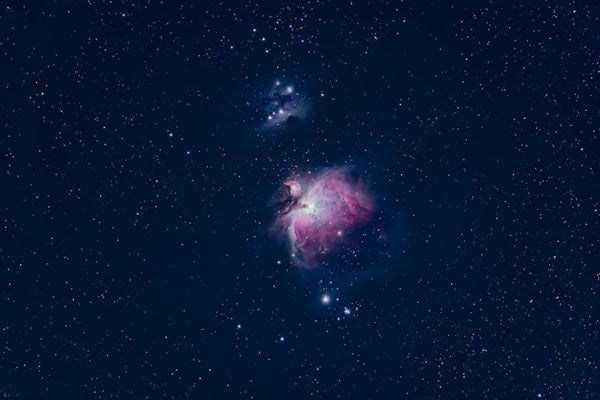
The best telescopes for deep space viewing
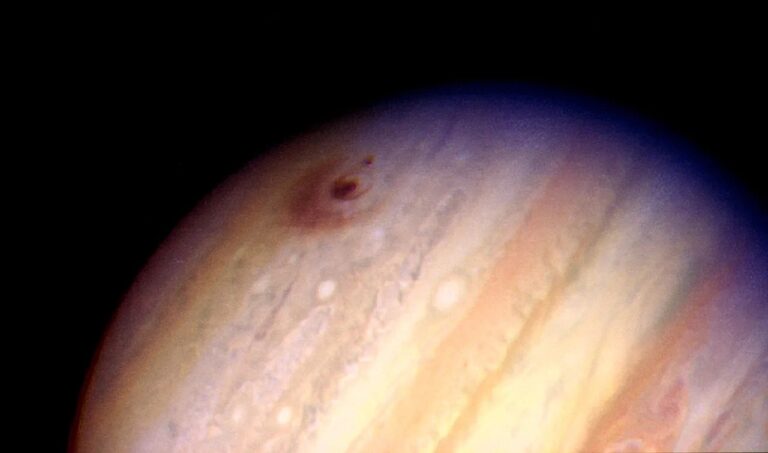
Comet Shoemaker-Levy 9: The backstory and its impact
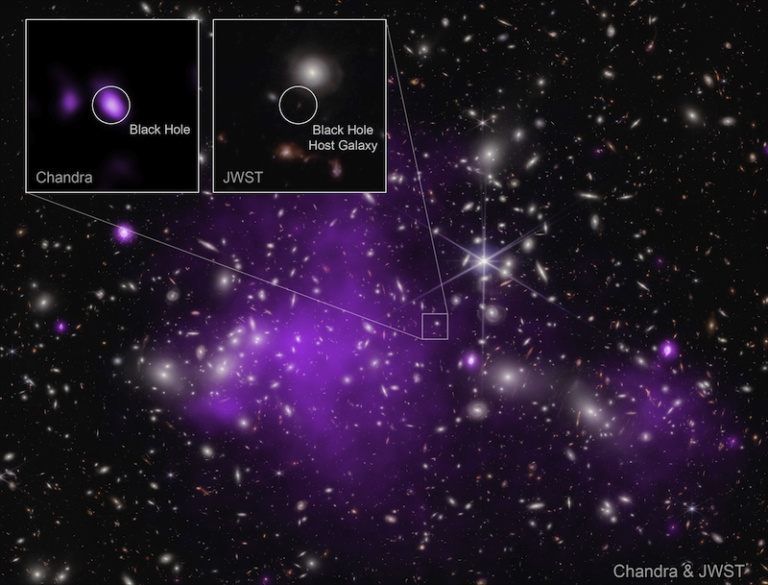
Astronomers say they’ve spotted the oldest, most distant black hole. How do they know this?

2024 Full Moon calendar: When to see the Full Moon and phases
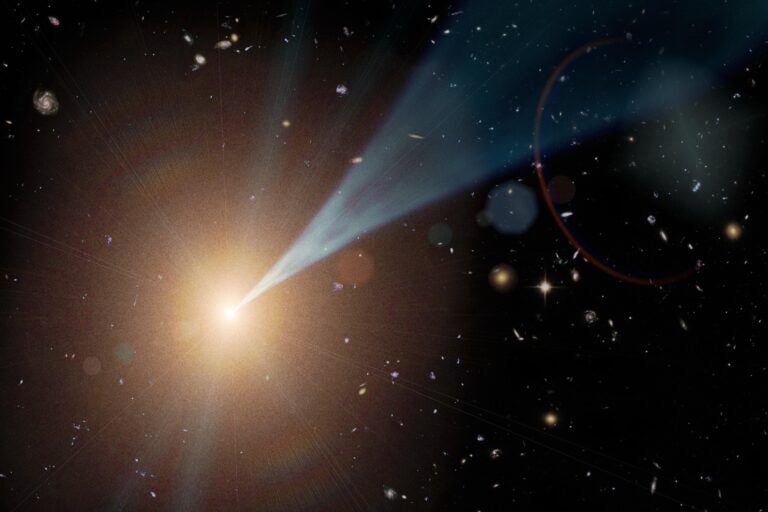
5 ways to achieve interstellar travel, ranked from least to most likely
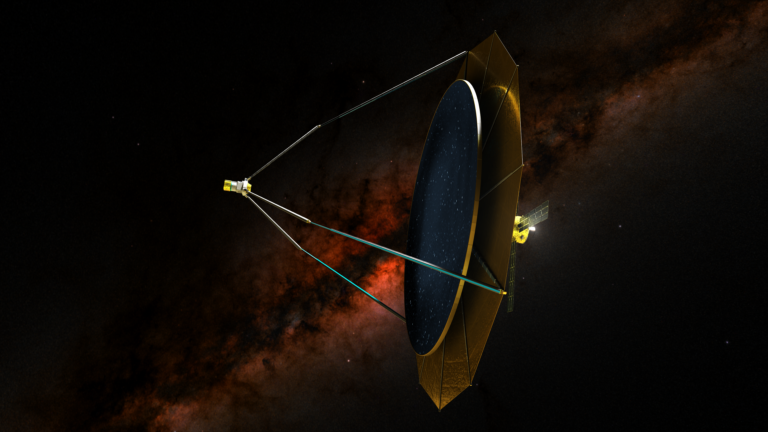
This NASA project could create staggeringly powerful telescopes

The history of astronomical almanacs
- History Classics
- Your Profile
- Find History on Facebook (Opens in a new window)
- Find History on Twitter (Opens in a new window)
- Find History on YouTube (Opens in a new window)
- Find History on Instagram (Opens in a new window)
- Find History on TikTok (Opens in a new window)
- This Day In History
- History Podcasts
- History Vault
Who determined the speed of light?
By: Elizabeth Nix
Updated: October 3, 2023 | Original: October 1, 2014
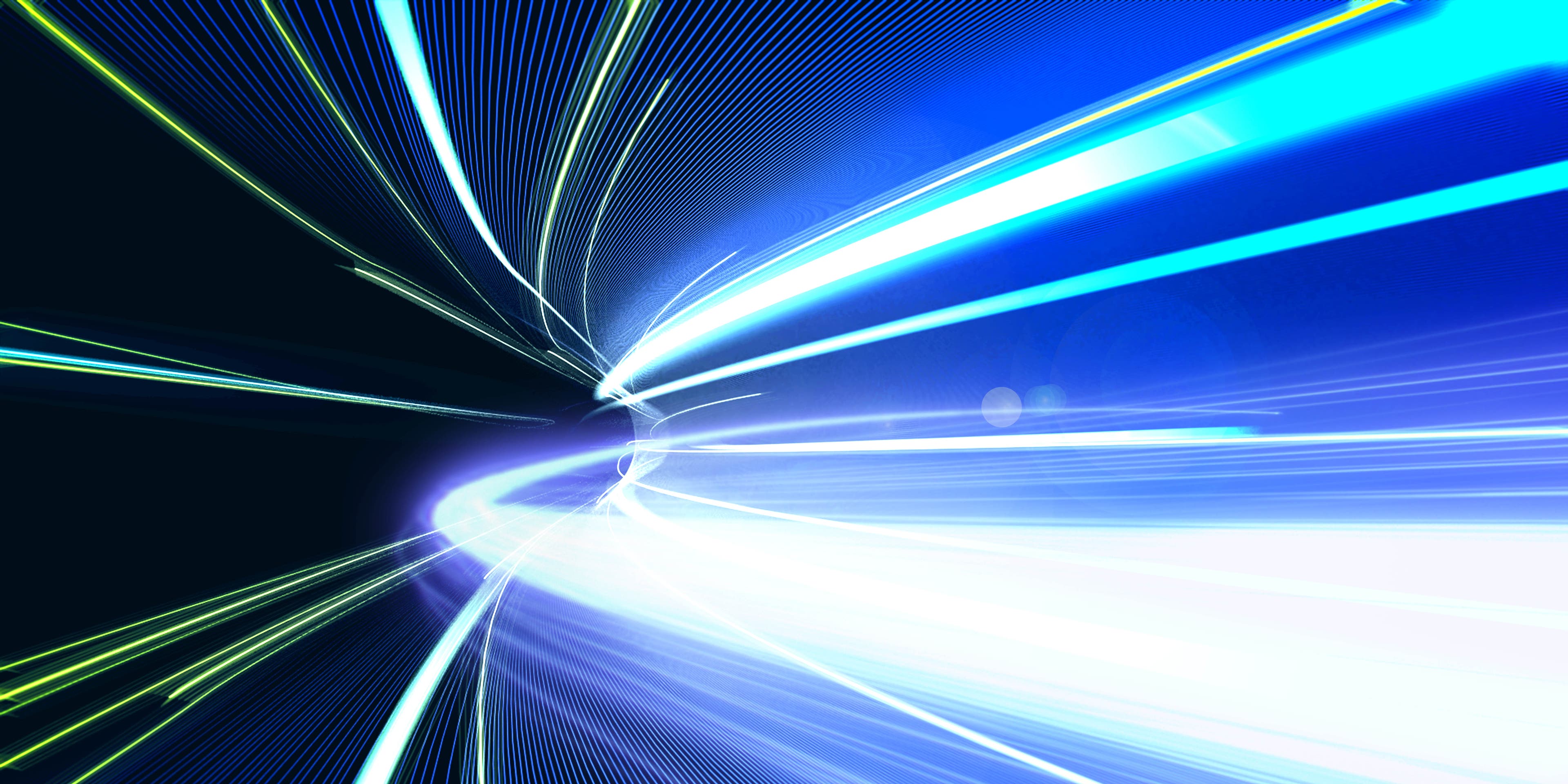
In ancient times, many scientists believed the speed of light was infinite and could travel any distance instantaneously. The Italian physicist Galileo Galilee was among the first to try to measure the speed of light. In the early 17th century, he devised an experiment in which two people with covered lanterns stood a known distance apart. One person uncovered his lantern and as soon as the other person saw the light, he uncovered his own lantern. Galileo attempted to record the time between lantern signals but was unsuccessful because the distance involved was too small and light simply moved too fast to be measured this way.
Around 1676, Danish astronomer Ole Roemer became the first person to prove that light travels at a finite speed. He studied Jupiter’s moons and noted that their eclipses took place sooner than predicted when Earth was nearer to Jupiter and happened later when Earth was farther away from Jupiter. Roemer reasoned this was the result of light moving at a finite speed; it took longer to make it to Earth when Jupiter was a greater distance away.
In the ensuing centuries, a number of other scientists worked to determine the speed of light and, using improved techniques, came up with increasingly accurate calculations. French physicist Hippolyte Fizeau is credited with making the first non-astronomical measurement, in 1849, using a method that involved sending light through a rotating toothed wheel then reflecting it back with a mirror located a significant distance away. One of the first precise calculations of light’s velocity was made in the 1920s by American physicist Albert Michelson, who carried out his research in the mountains of Southern California using an eight-sided rotating mirror apparatus.
In 1983, an international commission on weights and measures set the speed of light in a vacuum at the calculation we use today: 299,792,458 meters per second (186,282 miles per second)—a speed that could circle the equator 7.5 times in a single second.
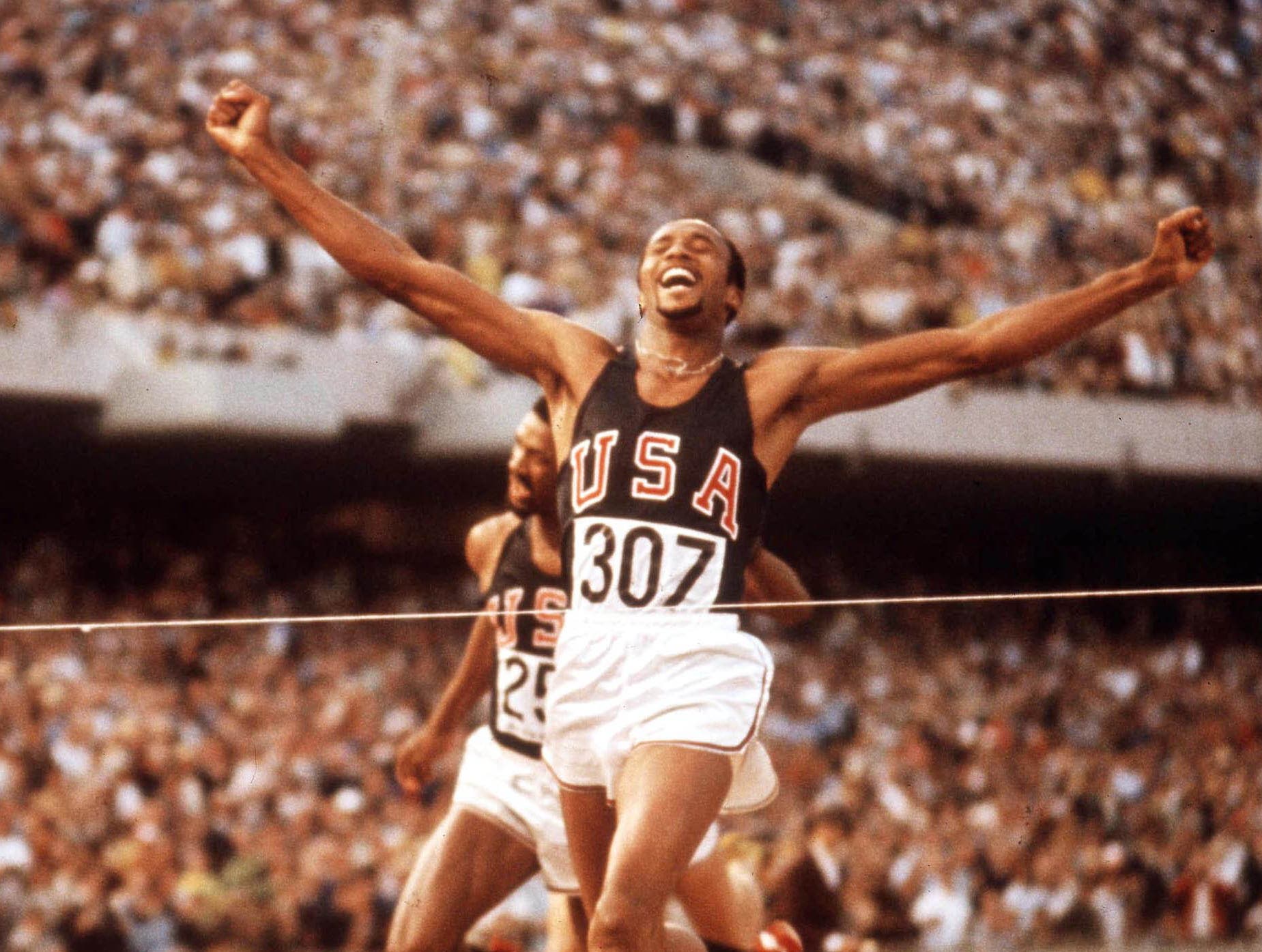
Sign up for Inside History
Get HISTORY’s most fascinating stories delivered to your inbox three times a week.
By submitting your information, you agree to receive emails from HISTORY and A+E Networks. You can opt out at any time. You must be 16 years or older and a resident of the United States.
More details : Privacy Notice | Terms of Use | Contact Us
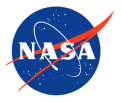
Suggested Searches
- Climate Change
- Expedition 64
- Mars perseverance
- SpaceX Crew-2
- International Space Station
- View All Topics A-Z
Humans in Space
Earth & climate, the solar system, the universe, aeronautics, learning resources, news & events.
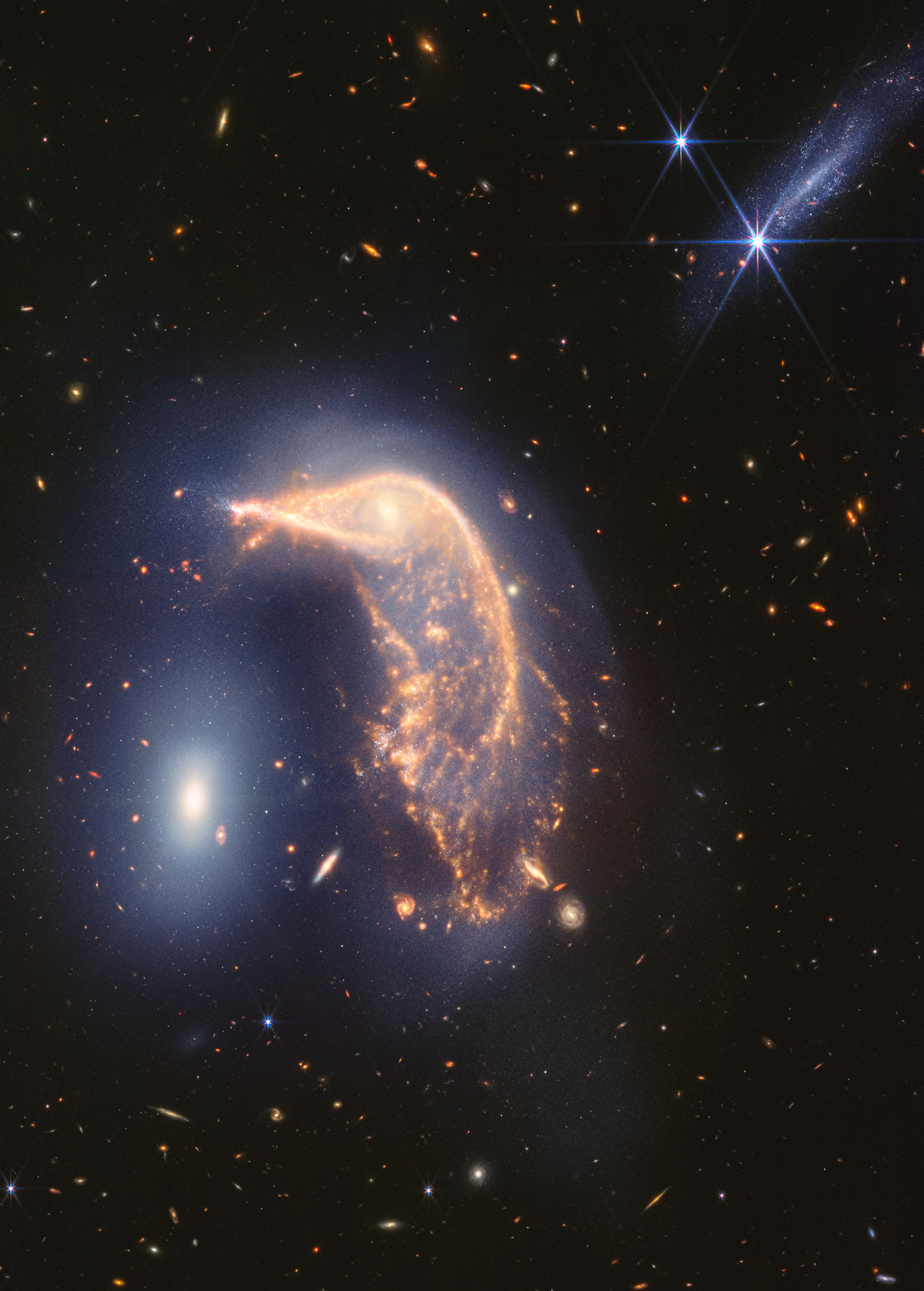
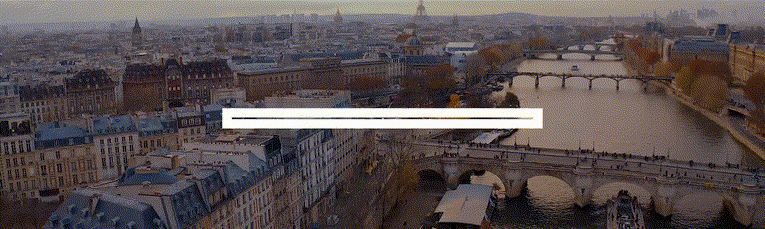
Vivid Portrait of Interacting Galaxies Marks Webb’s Second Anniversary
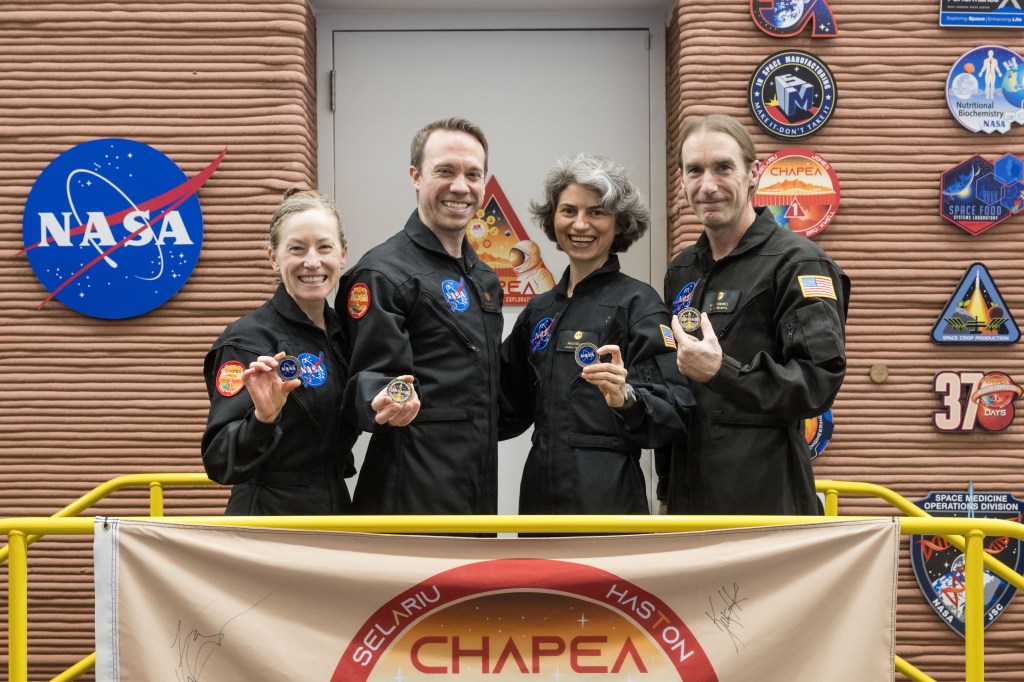
Voyagers of Mars: The First CHAPEA Crew’s Yearlong Journey

Surfing NASA’s Internet of Animals: Satellites Study Ocean Wildlife
- Search All NASA Missions
- A to Z List of Missions
- Upcoming Launches and Landings
- Spaceships and Rockets
- Communicating with Missions
- James Webb Space Telescope
- Hubble Space Telescope
- Why Go to Space
- Commercial Space
- Destinations
- Living in Space
- Explore Earth Science
- Earth, Our Planet
- Earth Science in Action
- Earth Multimedia
- Earth Science Researchers
- Pluto & Dwarf Planets
- Asteroids, Comets & Meteors
- The Kuiper Belt
- The Oort Cloud
- Skywatching
- The Search for Life in the Universe
- Black Holes
- The Big Bang
- Dark Energy & Dark Matter
- Earth Science
- Planetary Science
- Astrophysics & Space Science
- The Sun & Heliophysics
- Biological & Physical Sciences
- Lunar Science
- Citizen Science
- Astromaterials
- Aeronautics Research
- Human Space Travel Research
- Science in the Air
- NASA Aircraft
- Flight Innovation
- Supersonic Flight
- Air Traffic Solutions
- Green Aviation Tech
- Drones & You
- Technology Transfer & Spinoffs
- Space Travel Technology
- Technology Living in Space
- Manufacturing and Materials
- Science Instruments
- For Kids and Students
- For Educators
- For Colleges and Universities
- For Professionals
- Science for Everyone
- Requests for Exhibits, Artifacts, or Speakers
- STEM Engagement at NASA
- NASA's Impacts
- Centers and Facilities
- Directorates
- Organizations
- People of NASA
- Internships
- Our History
- Doing Business with NASA
- Get Involved
NASA en Español
- Aeronáutica
- Ciencias Terrestres
- Sistema Solar
- All NASA News
- Video Series on NASA+
- Newsletters
- Social Media
- Media Resources
- Upcoming Launches & Landings
- Virtual Events
- Sounds and Ringtones
- Interactives
- STEM Multimedia

15 Years Ago: STS-127 Delivers Japanese External Platform to Space Station

Tech Today: NASA’s Moonshot Launched Commercial Fuel Cell Industry
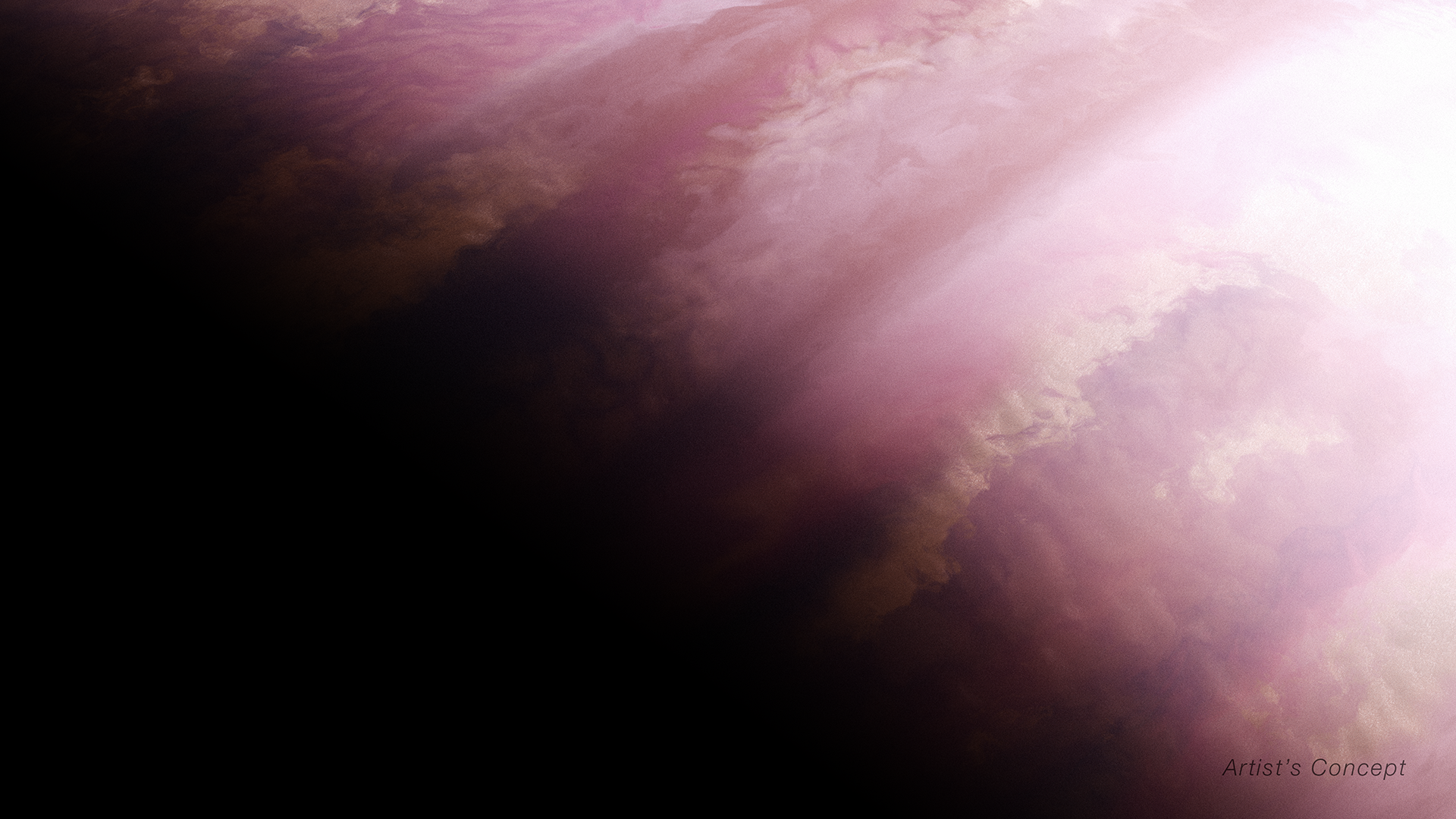
NASA’s Webb Investigates Eternal Sunrises, Sunsets on Distant World
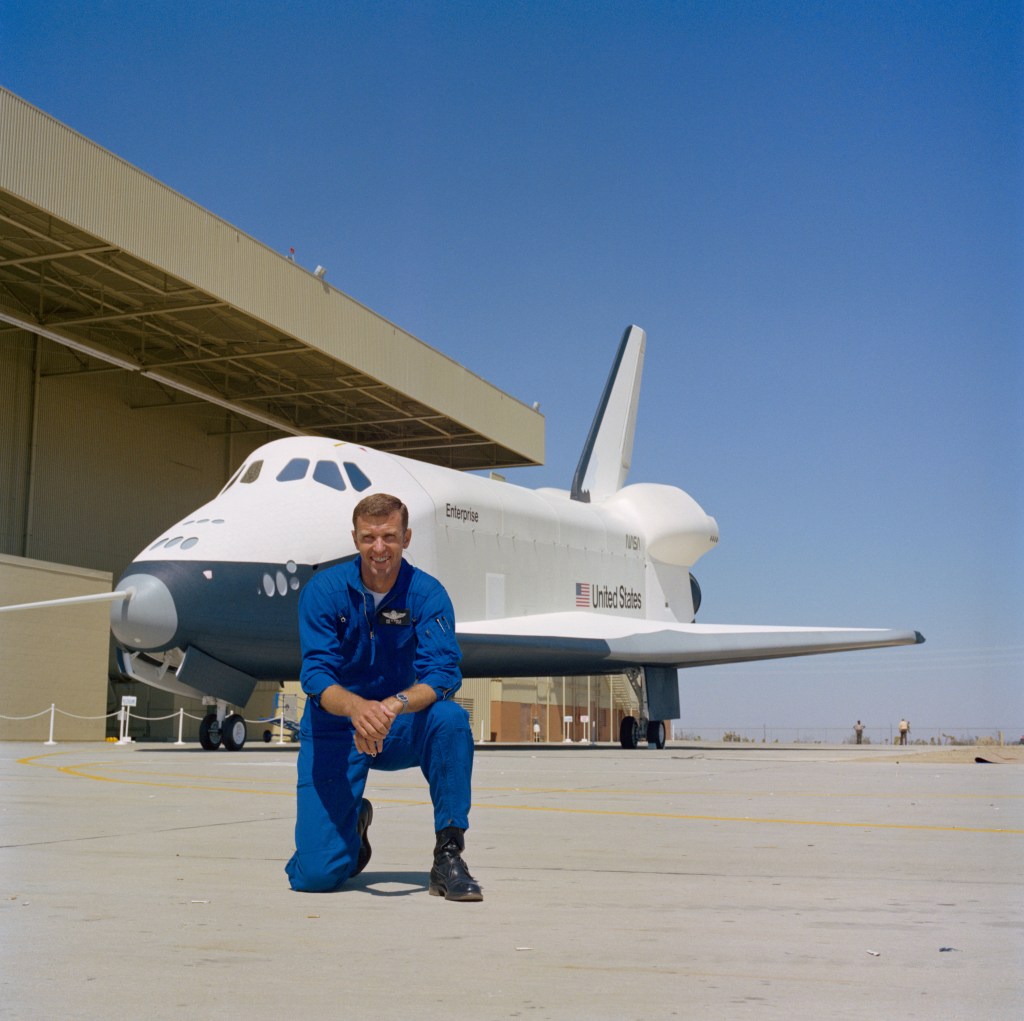
Remembering Joe Engle: Astronaut, Test Pilot, Legend
Joe h. engle oral history interviews.
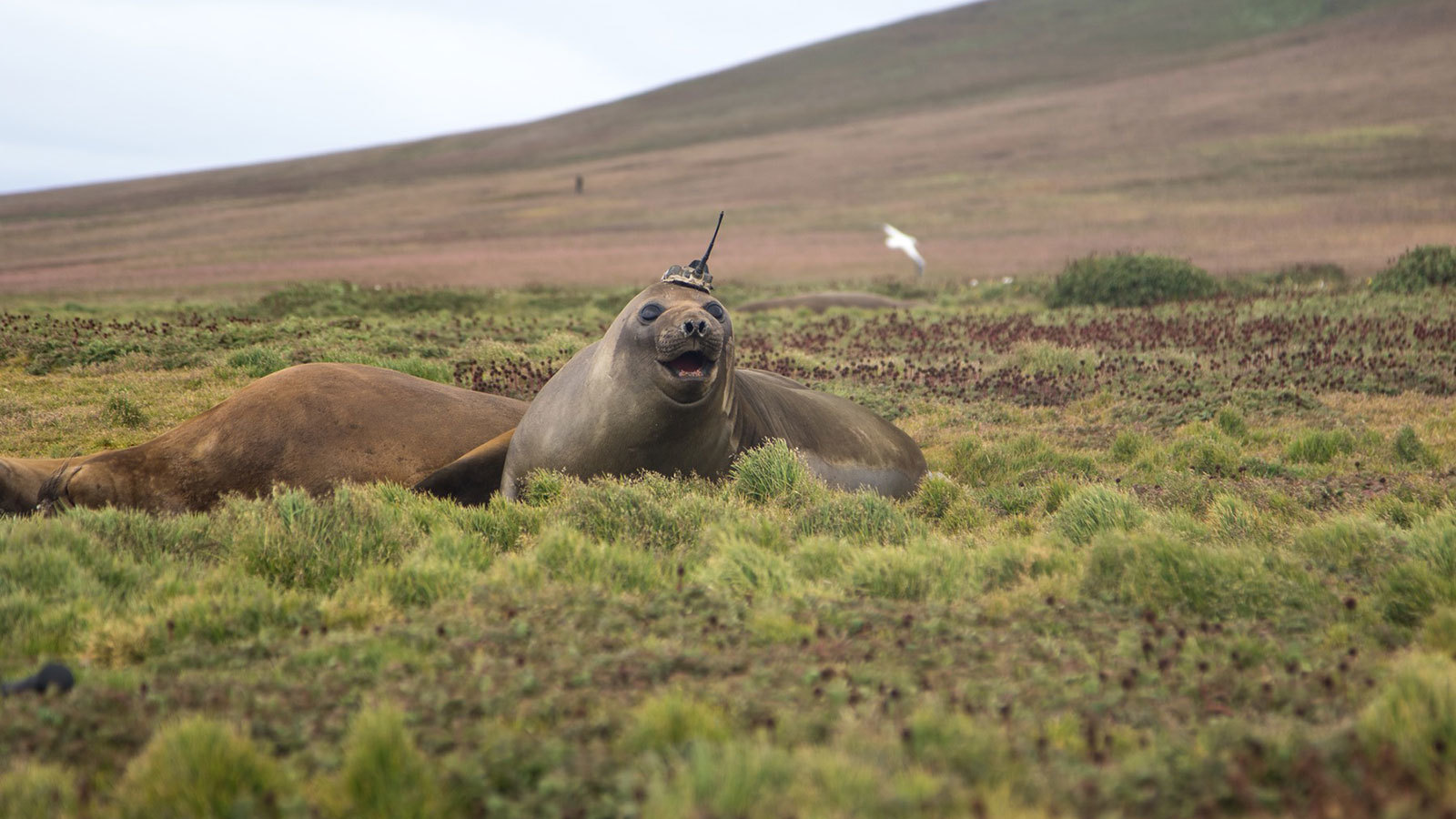
Amendment 28: Delay of Proposal Due Date for E.11 Consortium in Biological Sciences
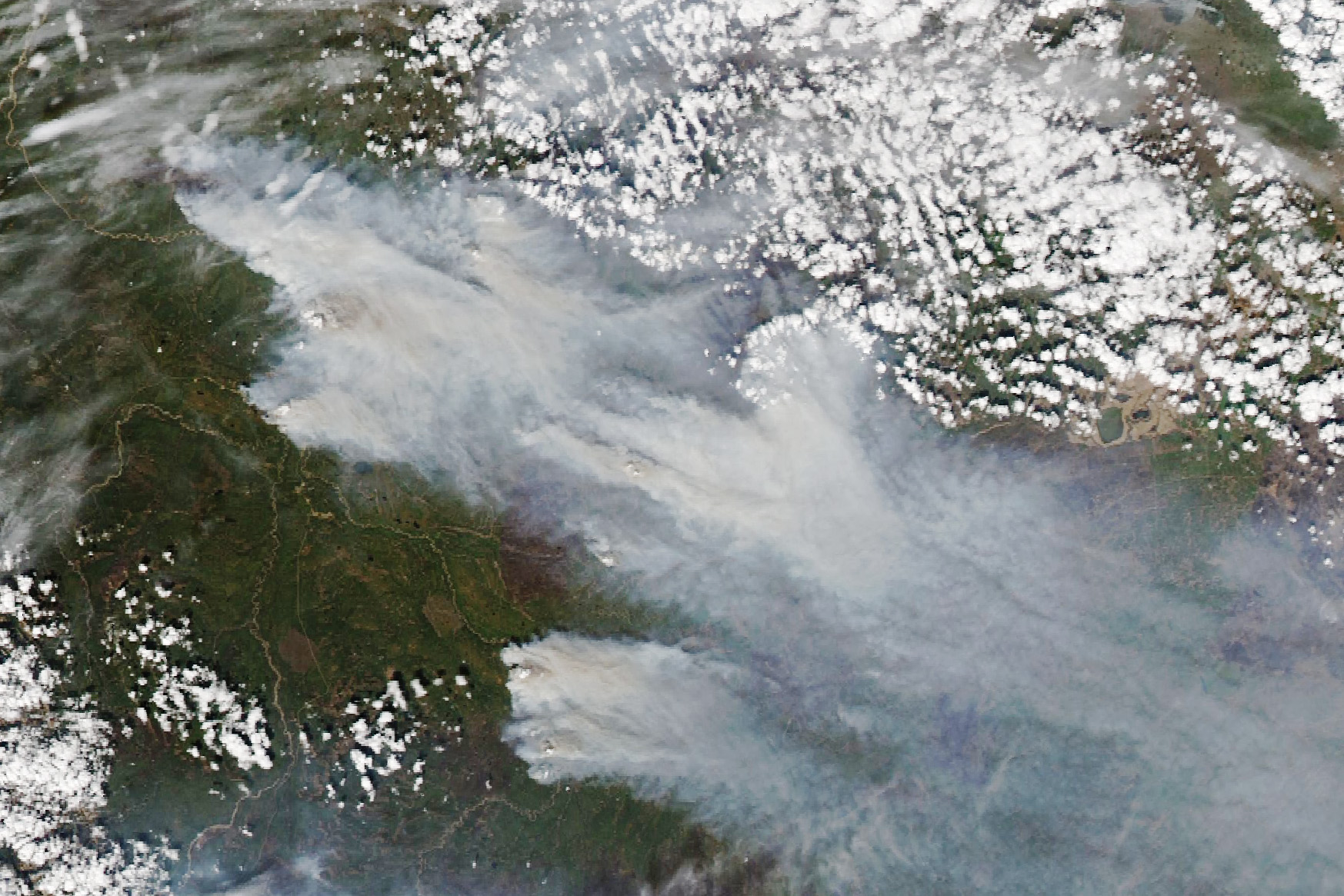
Amendment 26: A.44 Earth Action: Health and Air Quality Applied Sciences Team Proposal Due Date Delay.
Celebrate the heliophysics big year with free heliophysics and math webinars from nasa heat.
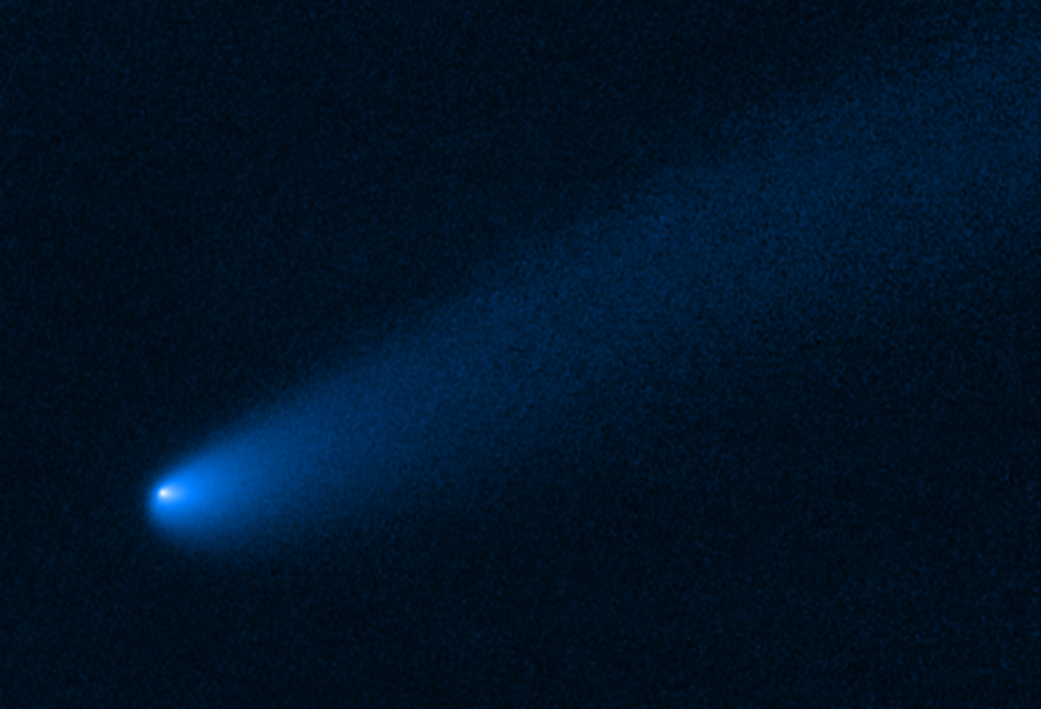
Amendment 25: New Opportunity: C.27 Lucy in the L4 Trojans Participating Scientist Program.
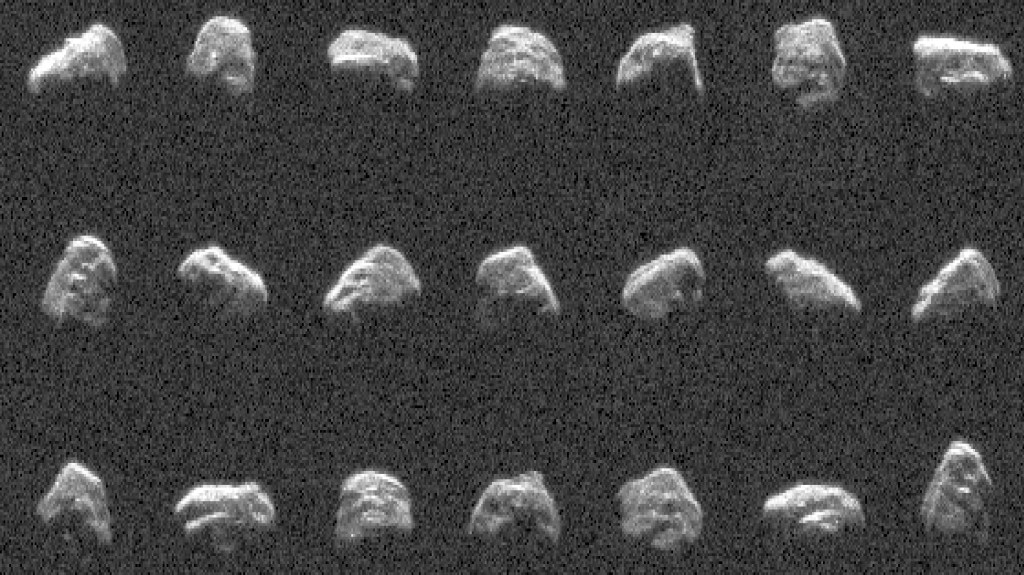
NASA’s Planetary Radar Tracks Two Large Asteroid Close Approaches
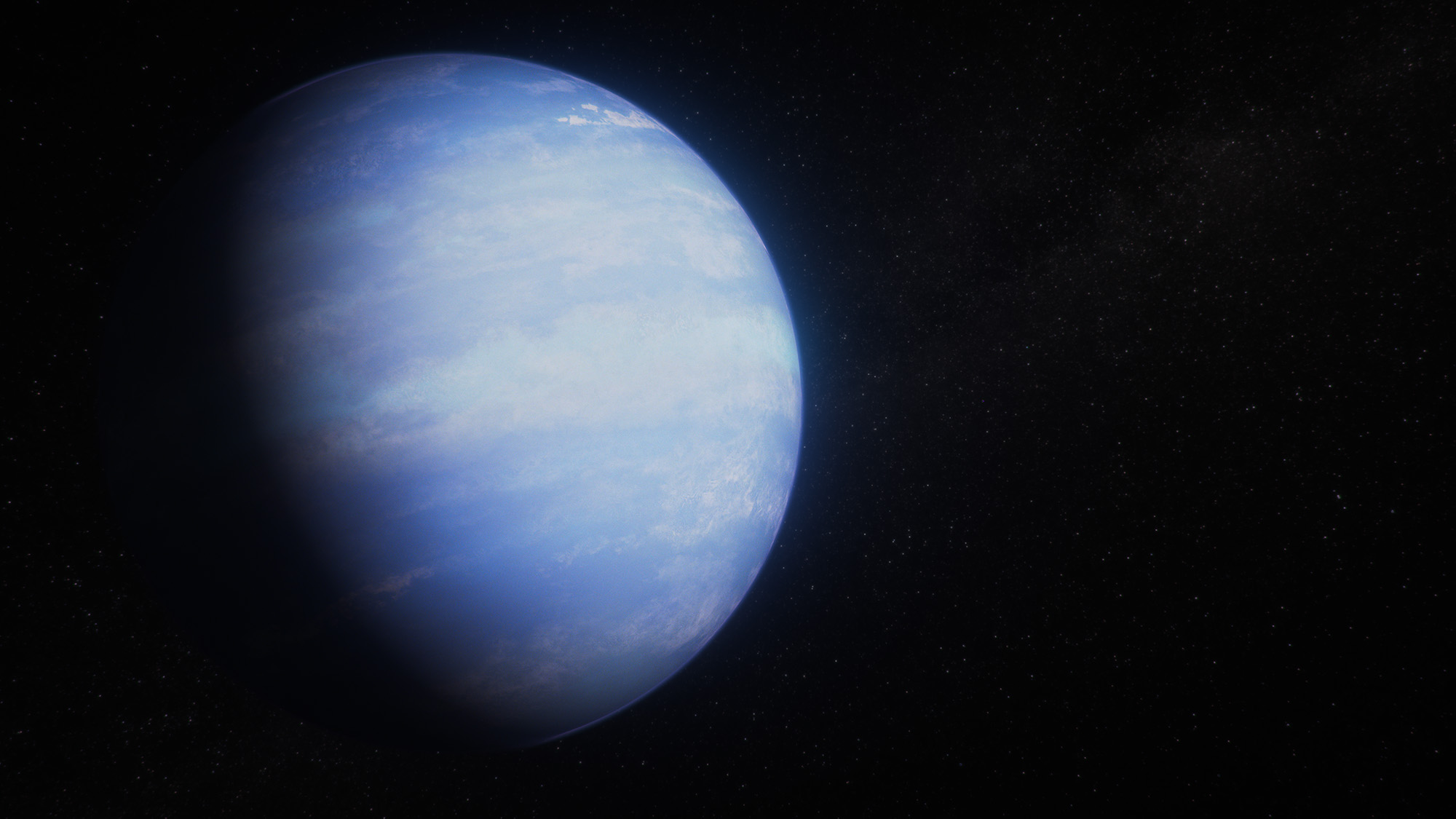
Amendment 29: New Opportunity D.20 Exoplanet Mass Measurement Program
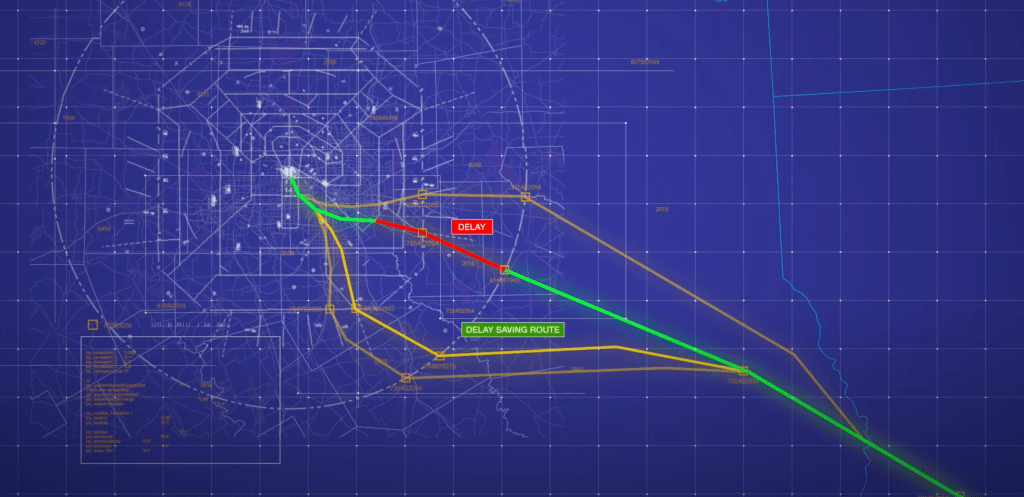
NASA Cloud-Based Platform Could Help Streamline, Improve Air Traffic

Bente Eegholm: Ensuring Space Telescopes Have Stellar Vision
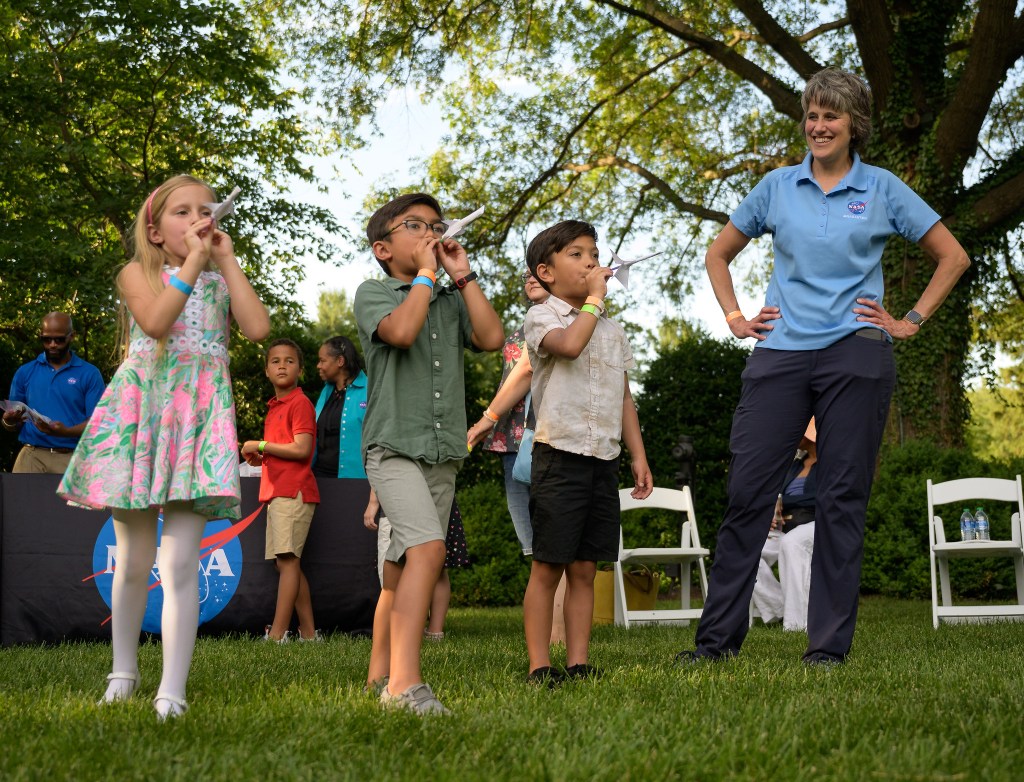
Slow Your Student’s ‘Summer Slide’ and Beat Boredom With NASA STEM

NASA Marshall Engineers Unveil Versatile, Low-cost Hybrid Engine Testbed

45 Years Ago: Skylab Reenters Earth’s Atmosphere
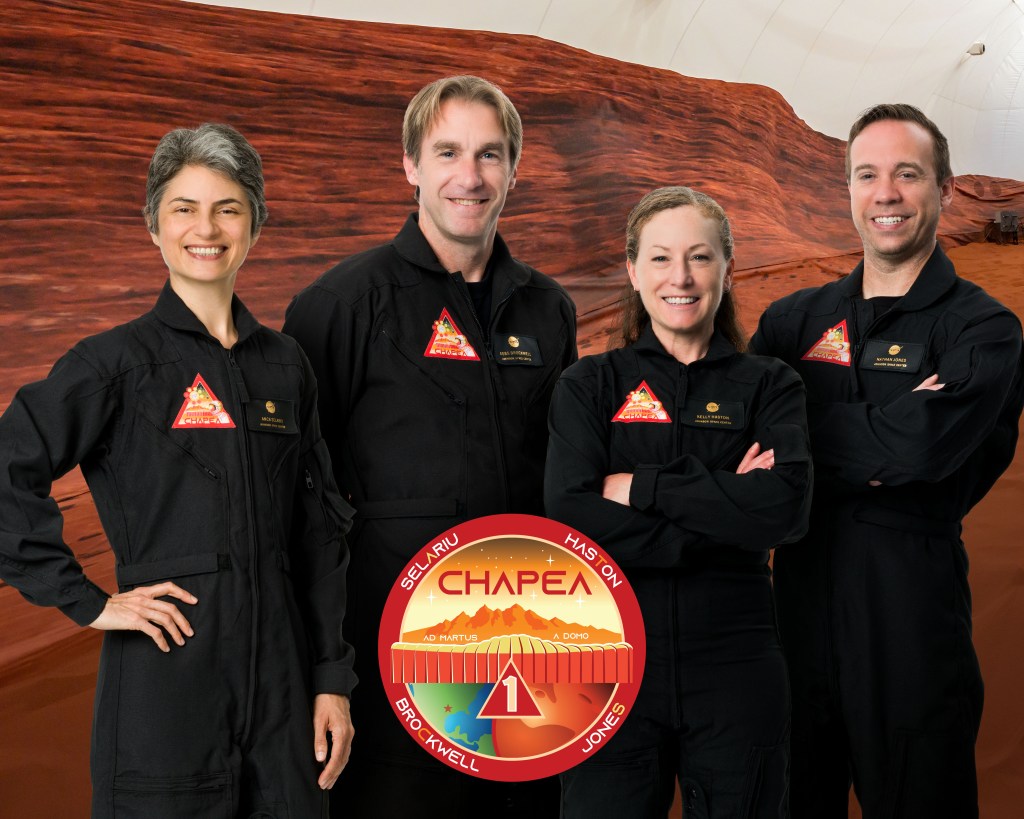
First Mars Crew Completes Yearlong Simulated Red Planet NASA Mission
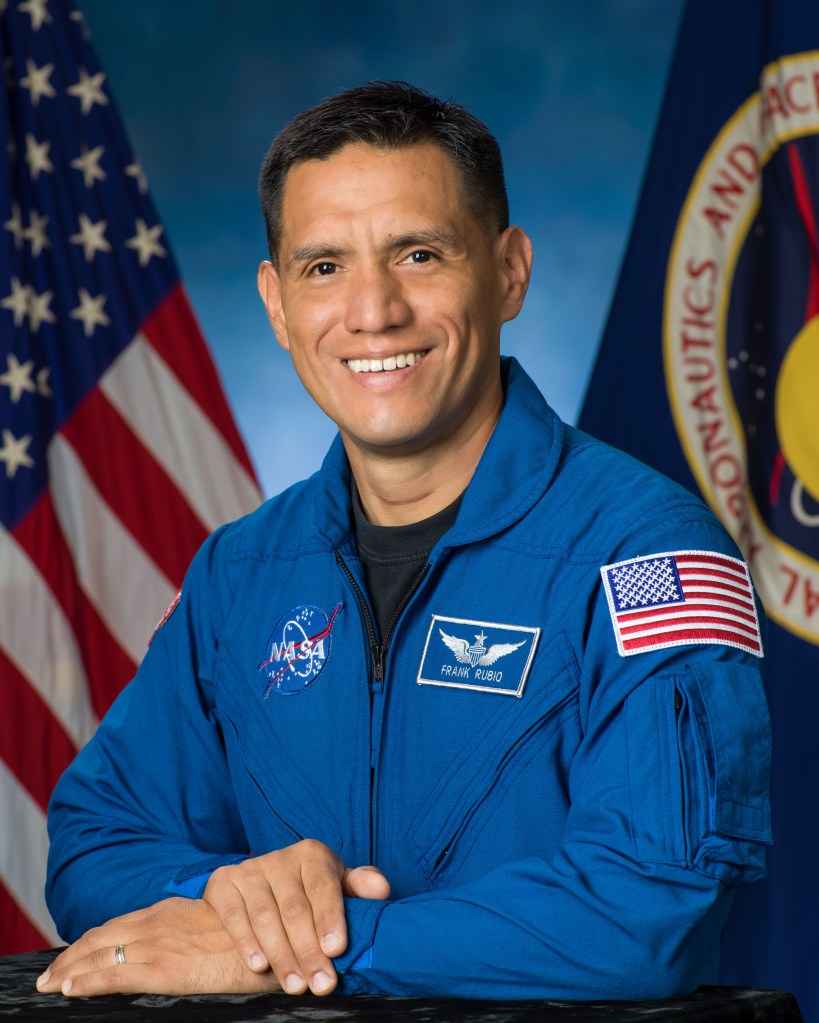
Astronauta de la NASA Frank Rubio
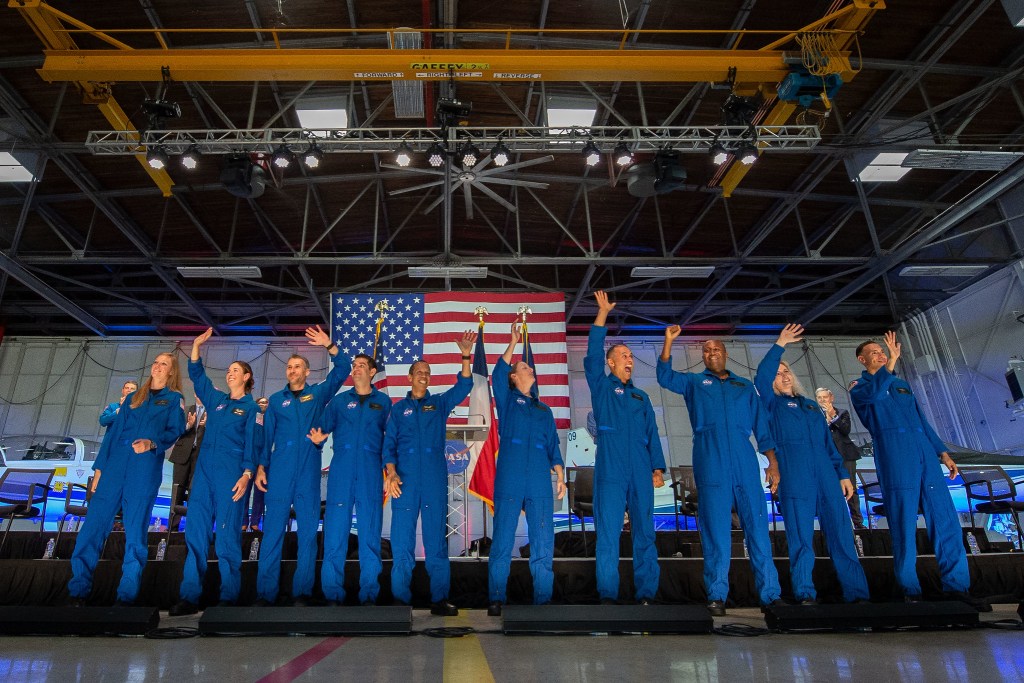
Diez maneras en que los estudiantes pueden prepararse para ser astronautas
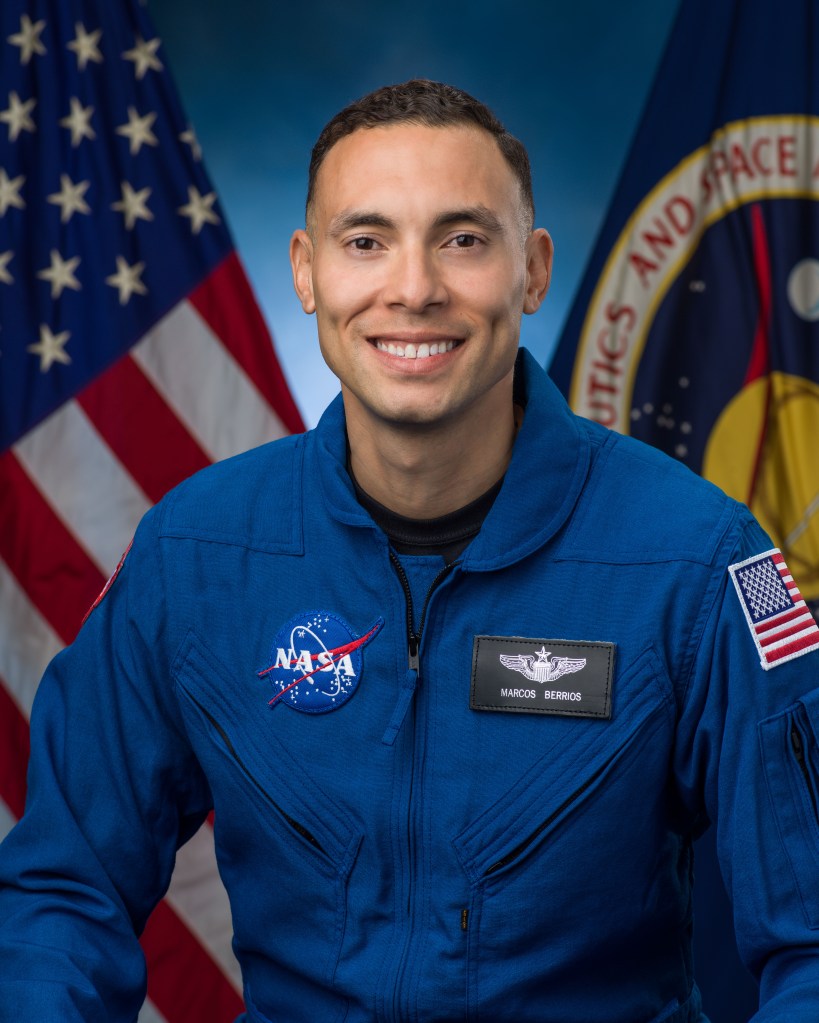
Astronauta de la NASA Marcos Berríos
Three ways to travel at (nearly) the speed of light.
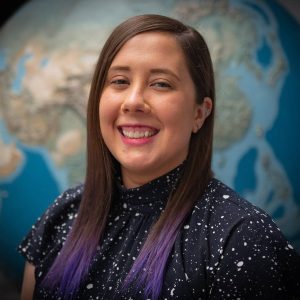
Katy Mersmann
1) electromagnetic fields, 2) magnetic explosions, 3) wave-particle interactions.
One hundred years ago today, on May 29, 1919, measurements of a solar eclipse offered verification for Einstein’s theory of general relativity. Even before that, Einstein had developed the theory of special relativity, which revolutionized the way we understand light. To this day, it provides guidance on understanding how particles move through space — a key area of research to keep spacecraft and astronauts safe from radiation.
The theory of special relativity showed that particles of light, photons, travel through a vacuum at a constant pace of 670,616,629 miles per hour — a speed that’s immensely difficult to achieve and impossible to surpass in that environment. Yet all across space, from black holes to our near-Earth environment, particles are, in fact, being accelerated to incredible speeds, some even reaching 99.9% the speed of light.
One of NASA’s jobs is to better understand how these particles are accelerated. Studying these superfast, or relativistic, particles can ultimately help protect missions exploring the solar system, traveling to the Moon, and they can teach us more about our galactic neighborhood: A well-aimed near-light-speed particle can trip onboard electronics and too many at once could have negative radiation effects on space-faring astronauts as they travel to the Moon — or beyond.
Here are three ways that acceleration happens.
Most of the processes that accelerate particles to relativistic speeds work with electromagnetic fields — the same force that keeps magnets on your fridge. The two components, electric and magnetic fields, like two sides of the same coin, work together to whisk particles at relativistic speeds throughout the universe.
In essence, electromagnetic fields accelerate charged particles because the particles feel a force in an electromagnetic field that pushes them along, similar to how gravity pulls at objects with mass. In the right conditions, electromagnetic fields can accelerate particles at near-light-speed.
On Earth, electric fields are often specifically harnessed on smaller scales to speed up particles in laboratories. Particle accelerators, like the Large Hadron Collider and Fermilab, use pulsed electromagnetic fields to accelerate charged particles up to 99.99999896% the speed of light. At these speeds, the particles can be smashed together to produce collisions with immense amounts of energy. This allows scientists to look for elementary particles and understand what the universe was like in the very first fractions of a second after the Big Bang.
Download related video from NASA Goddard’s Scientific Visualization Studio
Magnetic fields are everywhere in space, encircling Earth and spanning the solar system. They even guide charged particles moving through space, which spiral around the fields.
When these magnetic fields run into each other, they can become tangled. When the tension between the crossed lines becomes too great, the lines explosively snap and realign in a process known as magnetic reconnection. The rapid change in a region’s magnetic field creates electric fields, which causes all the attendant charged particles to be flung away at high speeds. Scientists suspect magnetic reconnection is one way that particles — for example, the solar wind, which is the constant stream of charged particles from the Sun — is accelerated to relativistic speeds.
Those speedy particles also create a variety of side-effects near planets. Magnetic reconnection occurs close to us at points where the Sun’s magnetic field pushes against Earth’s magnetosphere — its protective magnetic environment. When magnetic reconnection occurs on the side of Earth facing away from the Sun, the particles can be hurled into Earth’s upper atmosphere where they spark the auroras. Magnetic reconnection is also thought to be responsible around other planets like Jupiter and Saturn, though in slightly different ways.
NASA’s Magnetospheric Multiscale spacecraft were designed and built to focus on understanding all aspects of magnetic reconnection. Using four identical spacecraft, the mission flies around Earth to catch magnetic reconnection in action. The results of the analyzed data can help scientists understand particle acceleration at relativistic speeds around Earth and across the universe.
Particles can be accelerated by interactions with electromagnetic waves, called wave-particle interactions. When electromagnetic waves collide, their fields can become compressed. Charged particles bouncing back and forth between the waves can gain energy similar to a ball bouncing between two merging walls.
These types of interactions are constantly occurring in near-Earth space and are responsible for accelerating particles to speeds that can damage electronics on spacecraft and satellites in space. NASA missions, like the Van Allen Probes , help scientists understand wave-particle interactions.
Wave-particle interactions are also thought to be responsible for accelerating some cosmic rays that originate outside our solar system. After a supernova explosion, a hot, dense shell of compressed gas called a blast wave is ejected away from the stellar core. Filled with magnetic fields and charged particles, wave-particle interactions in these bubbles can launch high-energy cosmic rays at 99.6% the speed of light. Wave-particle interactions may also be partially responsible for accelerating the solar wind and cosmic rays from the Sun.
Download this and related videos in HD formats from NASA Goddard’s Scientific Visualization Studio
By Mara Johnson-Groh NASA’s Goddard Space Flight Center , Greenbelt, Md.
July 1849: Fizeau Publishes Results of Speed of Light Experiment
The speed of light is one of the most well-established values in physics, measured so accurately that the meter is now defined in terms of it. But before the 17th century, most scientists, including such giants as Johannes Kepler and Rene Descartes, considered the speed of light to be infinite, able to travel any distance instantaneously. Galileo Galilei was among the first to question this assumption and attempt to measure the speed of light experimentally.
By modern standards, Galileo’s methods were extremely crude. He stationed himself on one hilltop, and an assistant on a distant hilltop, each armed with a lamp that could be covered and uncovered at will. Galileo would uncover his lamp, and his assistant would do the same as soon as he observed the light from Galileo’s lamp. Knowing the distance between the two lamps, Galileo could measure how much time had elapsed between the two flashes to calculate the speed of light. Not surprisingly, his conclusion was rather vague and inconclusive: “If not instantaneous, it is extraordinarily rapid.” But he did conclude that light travels at least ten times faster than sound.
The first serious measurement of the speed of light occurred in 1676, when the Danish astronomer Ole Roemer observed the moons of Jupiter and noticed that their eclipses seemed to occur at different times, depending on the relative positions of Jupiter with respect to Earth, being late when Earth was far away, and early when Earth was closer to Jupiter. He correctly deduced that this effect wasn’t due to an actual shift in the moon’s orbits, but resulted because the light from those moons traveled a greater distance when Earth was farther away. He knew the accepted value for the diameter of Earth’s orbit at that time, and from that, he concluded that the speed of light was 240,000 kilometers per second.

Roemer’s measurement was still wide of the actual value, but it provided a useful baseline for future experiments. In 1728, an English physicist named James Bradley added his own findings to the accumulating body of knowledge, using stellar aberration to calculate the speed of light in a vacuum: in his case, 301,000 kilometers per second. The measurements were getting better. However, it would be another 100 years before a French scientist named Armand-Hippolyte-Louis Fizeau figured out how to measure the speed of light by means of a terrestrial experiment.
Born in Paris in 1819, Fizeau was the son of a physicist and professor of medicine, who left Fizeau a considerable fortune when he died. Free to pursue his interests without worrying about making a living, Fizeau focused on scientific research, initially intending to be a physician like his father, but ultimately choosing to study astronomy with Francois Arago at the Paris Observatory, where he no doubt learned of prior attempts to measure the speed of light using astronomical phenomena.
His scientific interests were quite varied, however. For instance, in 1839, he developed a fascination with Daguerrotype photography – then quite new – and teamed up with fellow French scientist Jean-Bernard-Leon Foucault in adapting the process to astronomy. It took 10 years, but the two men eventually took the first detailed photographs of the surface of the sun in 1845.
His work with Foucault inspired Fizeau to attempt his own measurement of the speed of light. He built an apparatus in which a cogwheel and a mirror were placed eight kilometers apart, and then sent pulses of light between them. He would rotate the cogwheel and observe how fast the beam of light traveled between the cogs of the wheel and the distant mirror, observing that if he spun the wheel very fast, the reflection back from the mirror was obscured because the light had struck one of the cogs.
Fizeau suggested that the amount of time it took the wheel to move the width of a single cog was equivalent to how long it took for the light beam to travel to the mirror and back again. Since he knew how fast the cogwheel was rotating, and the width of a single cog, as well as the distance to the mirror, Fizeau was able to calculate the speed of light, obtaining the value 313,300 kilometers per second. This was still roughly 5% too high.
Foucault improved on Fizeau’s apparatus slightly, replacing the cogwheel with a rotating mirror–hence it is now known as the Fizeau-Foucault Apparatus. Light was reflected at different angles as the mirror rotated. Since both the speed of rotation and the distance to the mirror were well established, it was possible to measure the difference between the angle of the light as it entered the apparatus and when it exited the setup, and calculate the speed of light from that. Foucault concluded in 1862 that the speed of light was 299,796 kilometers per second.
Fizeau’s contributions to science are not limited to this speed-of-light measurement. Subsequent experiments in which he measured how light traveled through flowing liquid resulted in a surprising discovery: the velocity of light doesn’t change as expected when the medium it is passing through is in motion. Scientists had already determined that light traveled at varying speeds through different mediums, but until Fizeau’s experiments, they believed that if a medium was moving, the speed of light would be obtained by simply adding the velocity of the medium to that of the light. His results implied a different formula, which would later be explained by Albert Einstein as the latter was developing his theory of special relativity.
Subsequent methods to measure the speed of light, of which Albert Michelson was a prominent practitioner, relied on wave interference. These methods became increasingly accurate with the advent of laser technology, and today, over 350 years after Galileo’s hilltop experiment, the speed of light’s value is defined to be 299,792.458 kilometers per second, according to a 1983 declaration by the 17th General Congress on Weights and Measures, thereby rendering the meter a derived quantity. It only took some 163 separate experiments involving more than 100 scientists – testament to the collaborative nature of the scientific enterprise.
Alan Chodos
Alan Chodos is the former editor of APS News.
Join your Society
If you embrace scientific discovery, truth and integrity, partnership, inclusion, and lifelong curiosity, this is your professional home.
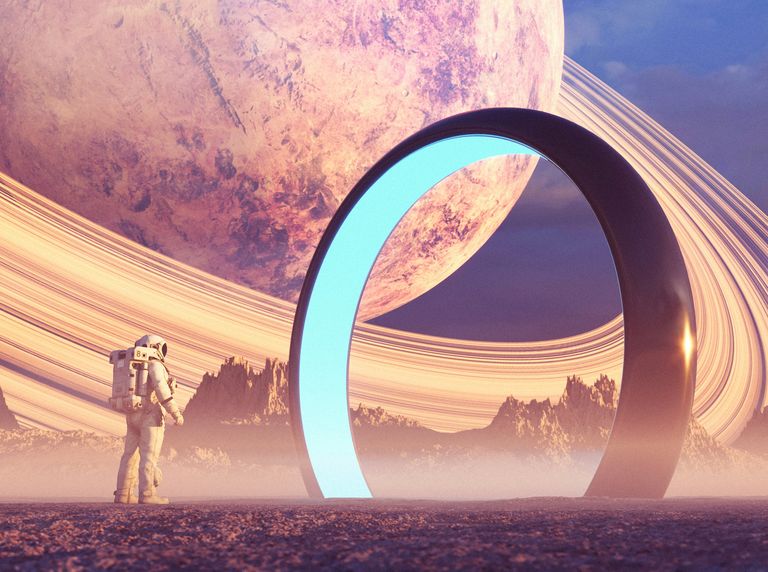
Scientists Believe Light Speed Travel Is Possible. Here’s How.
A functioning warp drive would allow humans to reach the far ends of the cosmos in the blink of an eye.
White and his team in LSI’s Houston laboratory were conducting research for the Defense Advanced Research Projects Agency, or DARPA, and had set up these particular experiments to study the energy densities within Casimir cavities, the mysterious spaces between microscopic metal plates in a vacuum. The data plot indicated areas of diminished energy between the plates, which caused them to push toward each other as if trying to fill the void. This is known as negative vacuum energy density, a phenomenon in quantum mechanics called, appropriately enough, the Casimir effect . It’s something that’s helping scientists understand the soupy physics of microscale structures, which some researchers hope can be applied to energy applications that are more practical, such as circuits and electromechanical systems.
But White noticed that the pattern of negative vacuum energy between the plates and around tiny cylindrical columns that they’d inserted in the space looked familiar. It precisely echoed the energy pattern generated by a type of exotic matter that some physicists believe could unlock high-speed interstellar travel. “We then looked, mathematically, at what happens if we placed a one-micron sphere inside of a four-micron cylinder under the same conditions, and found that this kind of structure could generate a little nanoscale warp bubble encapsulating that central region,” White explains.
That’s right—a warp bubble. The essential component of a heretofore fictional warp drive that has for decades been the obsession of physicists, engineers, and sci-fi fans. Warp drive, of course, is the stuff of Star Trek legend, a device enclosed within a spacecraft that gives the mortals aboard the ability to rip around the cosmos at superhuman speed. To the lay sci-fi fan, it’s a “black box”—a convenient, completely made-up workaround to avoid the harsh realities of interstellar travel. However, after decades of speculation, research, and experimentation, scientists believe a warp drive could actually work.
To emphasize: White didn’t actually make a warp bubble. But the data from his study led to an aha moment: For the first time, a buildable warp bubble showed promise of success.
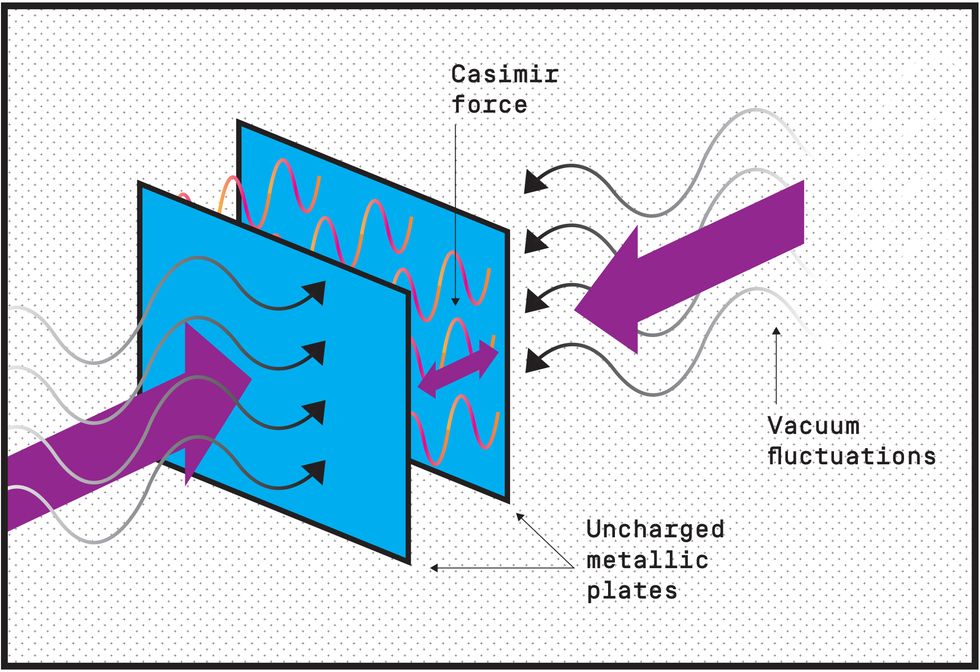
Warp technology’s core science is surprisingly sound. Though the specific mechanics of an actual device haven’t been fully unpacked, the math points toward feasibility. In short, a real-life warp drive would use massive amounts of energy, which can come in the form of mass, to create enough gravitational pull to distort spacetime in a controlled fashion, allowing a ship to speed along inside a self-generated bubble that itself is able to travel at essentially any speed. Warp drives popped up in fiction intermittently for several decades before Star Trek creator Gene Roddenberry plugged one into the USS Enterprise in 1966. But Miguel Alcubierre, PhD, a Mexican theoretical physicist and professed Star Trek enthusiast, gave the idea real-world legs when he released a paper in 1994 speculating that such a drive was mathematically possible. It was the first serious treatment of a warp drive’s feasibility, and it made headlines around the world. His breakthrough inspired more scientists to nudge the theoretical aspects of warp drive toward concrete, practical applications.
“I proposed a ‘geometry’ for space that would allow faster-than-light travel as seen from far away, essentially expanding space behind the object we want to move and contracting it in front,” Alcubierre says. “This forms a ‘bubble’ of distorted space, inside of which an object—a spaceship, say—could reside.”
Physicists tend to speak in relative terms. By injecting the sly qualifier “as seen from far away,” Alcubierre might sound like he’s describing the galactic equivalent of an optical illusion —an effect perhaps similar to driving past a truck going the opposite direction on the highway when you’re both going 60 miles an hour. Sure feels like a buck-twenty, doesn’t it? But the A-to-B speed is real; the warp effect simply shortens the literal distance between two points. You’re not, strictly speaking, moving faster than light. Inside the bubble, all appears relatively normal, and light moves faster than you are, as it should. Outside the bubble, however, you’re haulin’ the mail.
.css-1i6271r{margin:0rem;font-size:1.625rem;line-height:1.2;font-family:UnitedSans,UnitedSans-roboto,UnitedSans-local,Helvetica,Arial,Sans-serif;padding:0.9rem 1rem 1rem;}@media(max-width: 48rem){.css-1i6271r{font-size:1.75rem;line-height:1;}}@media(min-width: 48rem){.css-1i6271r{font-size:1.875rem;line-height:1;}}@media(min-width: 64rem){.css-1i6271r{font-size:2.25rem;line-height:1;}}.css-1i6271r em,.css-1i6271r i{font-style:italic;font-family:inherit;}.css-1i6271r b,.css-1i6271r strong{font-family:inherit;font-weight:bold;} THOUGH THE SPECIFIC MECHANICS OF AN ACTUAL DEVICE HAVEN’T BEEN UNPACKED, THE MATH POINTS TOWARD FEASIBILITY.
Alcubierre’s proposal had solved one of the initial hurdles to achieving warp speeds: The very idea clashes with Einstein’s long-accepted theory of general relativity, which states that nothing can travel faster than the speed of light, but it doesn’t preclude space itself from traveling faster than that. In fact, scientists speculate that the same principles explain the rapid expansion of the universe after the Big Bang .
While concluding that warp speed was indeed possible, Alcubierre also found that it would require an enormous amount of energy to sustain the warp bubble. He theorized that negative energy—the stuff hinted at by White’s experimentation with Casimir cavities—could be a solution. The only problem is that no one has yet proved that negative energy is real. It’s the unobtanium of our spacefaring imaginations, something researchers only believe to exist. In theory, however, this unknown matter may be sufficiently powerful that future warp drive designers could channel it to contract spacetime around it. In conceptual drawings of warp-capable spacecraft , enormous material rings containing this energy source surround a central fuselage. When activated, it warps spacetime around the entire ship. The more intense the warping, the faster the warp travel is achieved.
Of course, it’s not that simple. Physicist José Natário, PhD, a professor at the Instituto Superior Técnico in Lisbon, wrote his own influential paper about the mathematical feasibility of warp drives in 2001. However, he is concerned about practical conundrums, like the amount of energy required. “You need to be able to curve spacetime quite a lot in order to do this,” he says. “We’re talking about something that would be much, much more powerful than the sun.”
Alcubierre is similarly skeptical that his theoretical ideas might ever be used to develop a working warp drive. “In order to have a bubble about 100 meters wide traveling at precisely the speed of light, you would need about 100 times the mass of the planet Jupiter converted into negative energy, which of course sounds absurd,” he says. By that standard, he concludes, a warp drive is very unlikely.
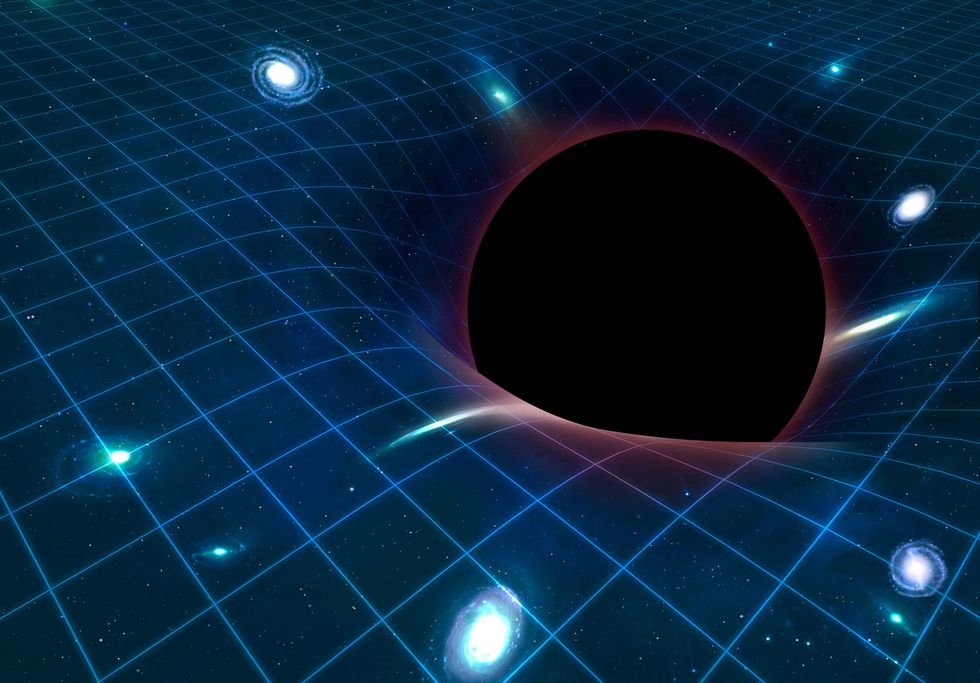
Physicists love a challenge, though. In the 29 years since Alcubierre published his paper, other scientists have wrestled with the implications of the work, providing alternative approaches to generating the energy using more accessible power sources, finding oblique entry points to the problem, and batting ideas back and forth in response to one another’s papers. They use analogies involving trampolines , tablecloths, bowling balls, balloons, conveyor belts, and music to explain the physics.
They even have their own vocabulary. It’s not faster-than-light travel; it’s superluminal travel, thank you. Then there’s nonphysical and physical—a.k.a. the critical distinction between theoretical speculation and something that can actually be engineered. (Pro tip: We’re aiming for physical here, folks.) They do mention Star Trek a lot, but never Star Wars . Even the scruffiest-looking nerf herder knows that the ships in Star Wars use hyperdrive, which consumes fuel, rather than warp drives, which don’t use propulsive technology but instead rely on, well, warping. They’re also vague about details like what passengers would experience, what gravity is like on board since you’re carrying around boatloads of energy, and what would happen if someone, say, jumped out of the ship while warping. (A speculative guess: Nothing good.)
Such research isn’t typically funded by academic institutions or the DARPAs and NASAs of the world, so much of this work occurs in the scientists’ spare time. One such scientist and Star Trek enthusiast is physicist Erik Lentz, PhD. Now a researcher at Pacific Northwest National Laboratory in Richland, Washington, Lentz was doing postdoctoral work at Göttingen University in Germany when, amid the early, isolated days of the pandemic, he mulled the idea of faster-than-light travel. He published a paper in 2021 arguing that warp drives could be generated using positive energy sources instead of the negative energy that Alcubierre’s warp drive seemed to require.
“There are a number of barriers to entry to actually being able to build a warp drive,” Lentz says. “The negative energy was the most obvious, so I tried to break that barrier down.”
He explored a new class of solutions in Einstein’s general relativity while focusing on something called the weak-energy condition, which, he explains, tracks the positivity of energy in spacetime. He hit upon a “soliton solution”—a wave that maintains its shape and moves at a constant velocity—that could both satisfy the energy-level challenge and travel faster than light. Such a warp bubble could travel along using known energy sources, though harnessing those at the levels needed are still far beyond our capabilities. The next step, he notes, may be bringing the energy requirements for a warp drive to within the range of a nuclear fusion reactor.
A fusion-powered device could theoretically travel to and from Proxima Centauri , Earth’s nearest star, in years instead of decades or millennia, and then go faster and faster as power sources improve. Current conventional rocket technology, on the other hand, would take 50,000 years just for a one-way trip—assuming, of course, there was an unlimited fuel supply for those engines.
“IF YOU COLLIDE WITH SOMETHING ON YOUR PATH, IT WOULD ALMOST CERTAINLY BE CATASTROPHIC.”
Like Alcubierre’s original thesis, Lentz’s paper had a seismic impact on the warp drive community, prompting yet another group of scientists to dig into the challenge. Physicist Alexey Bobrick and technology entrepreneur Gianni Martire have been particularly prolific. In 2021, they released a paper theorizing that a class of subliminal warp drives, traveling at just a fraction of light speed, could be developed from current scientific understanding. While that paper essentially argued that it’s perfectly acceptable to walk before you can run, they followed it up with another theory earlier this year that describes how a simulated black hole , created using sound waves and glycerin and tested with a laser beam, could be used to evaluate the levels of gravitational force needed to warp spacetime. The duo coded that breakthrough into a public app that they hope will help more quickly push theoretical ideas to practical ones. Though the team is waiting for the technology to clear a peer review stage before releasing details, the app is essentially a simulator that allows scientists to enter their warp-speed equations to validate whether they’re practical.
“When somebody publishes a warp metric for the first time, people say, ‘Okay, is your metric physical?’” Martire says. The answer to that question—whether the metric has practical potential or is strictly theoretical—is hard to establish given the challenges of testing these hypotheses. That determination could take six to eight months. “Now we can tell you within seconds, and it shows you visually how off you are or how close you are,” he says.
While useful, the app will speed up the preliminary math only for future researchers. Galaxy-sized challenges remain before we ever experience turbocharged interstellar travel. Alcubierre worries in particular about what may happen near the walls of the warp bubble. The distortion of space is so violent there, he notes, that it would destroy anything that gets close. “If you collide with something on your path, it would almost certainly be catastrophic,” he says.
Natário mulls even more practical issues, like steering and stopping. “It’s a bubble of space, that you’re pushing through space,” he says. “So, you’d have to tell space ... to curve in front of your spaceship.” But therein lies the problem: You can’t signal to the space in front of you to behave the way you want it to.
His opinion? Superluminal travel is impossible. “You need these huge deformations that we have no idea how to accomplish,” Natário says. “So yes, there has been a lot of effort toward this and studying these weird solutions, but this is all still completely theoretical, abstract, and very, very, very, very far from getting anywhere near a practical warp drive.” That’s “very” to the power of four, mind you—each crushing blow pushing us exponentially, excruciatingly further and further away from our yearned-for superluminal lives.
Ultimately, the pursuit of viable high-speed interstellar transportation also points to a more pressing terrestrial challenge: how the scientific community tackles ultra-long-term challenges in the first place. Most of the research so far has come from self-starters without direct funding, or by serendipitous discoveries made while exploring often unrelated research, such as Dr. White’s work on Casimir cavities.
Many scientists argue that we’re in a multi-decade period of stagnation in physics research, and warp drive—despite its epic time horizons before initial research leads to galaxy-spanning adventures—is somewhat emblematic of that stagnation. Sabine Hossenfelder, a research fellow at the Frankfurt Institute for Advanced Studies and creator of the YouTube channel Science Without the Gobbledygook , noted in a 2020 blog post that physics research has drifted away from frequent, persistent physical experimentation to exorbitant infusions of cash into relatively few devices. She writes that with fewer experiments, serendipitous discoveries become increasingly unlikely. Without those discoveries, the technological progress needed to keep experiments economically viable never materializes.
When asked whether this applied equally to warp drive, Hossenfelder sees a faint but plausible connection. “Warp drives are an idea that is not going to lead to applications in the next 1,000 years or so,” she says. “So they don’t play a big role in that one way to another. But when it comes to the funding, you see some overlap in the problems.”
So, despite all the advances, the horizon for a warp drive remains achingly remote. That hasn’t fazed the scientists involved, though. A few years ago, while teaching in France, White visited the Strasbourg Cathedral with his wife. While admiring its 466-foot-tall spire, he was struck by the fact that construction began in 1015 but didn’t wrap up until 1439—a span of 424 years. Those who built the basement had no chance of ever seeing the finished product, but they knew they had to do their part to aid future generations. “I don’t have a crystal ball,” White says. “I don’t know what the future holds. But I know what I need to be doing right now.”
Eric Adams is a writer and photographer who focuses on technology, transportation, science, travel, and other subjects for a wide range of outlets, including Wired, The Drive, Gear Patrol, Men's Health, Popular Science, Forbes, and others.
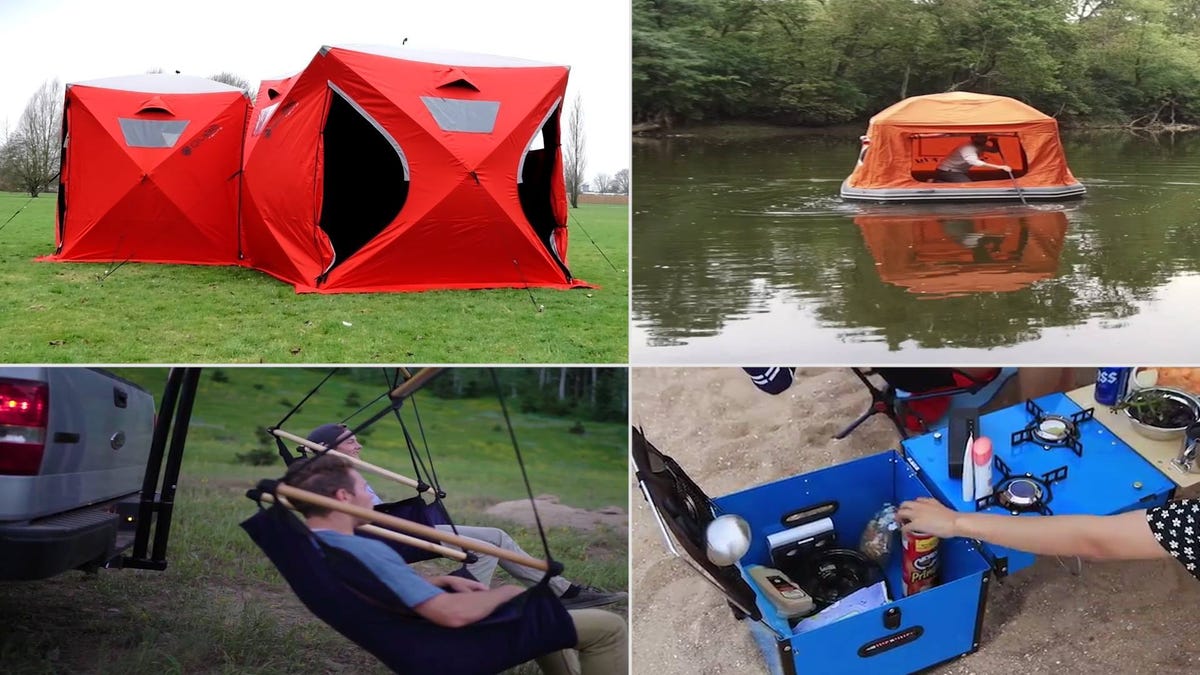
.css-cuqpxl:before{padding-right:0.3125rem;content:'//';display:inline;} Pop Mech Pro .css-xtujxj:before{padding-left:0.3125rem;content:'//';display:inline;}
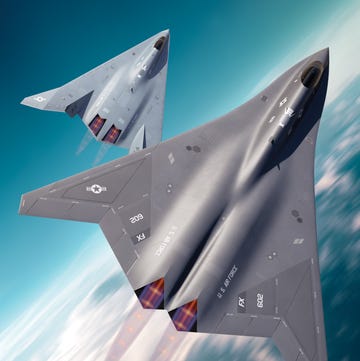
Humans Could Acquire a New Form of Consciousness
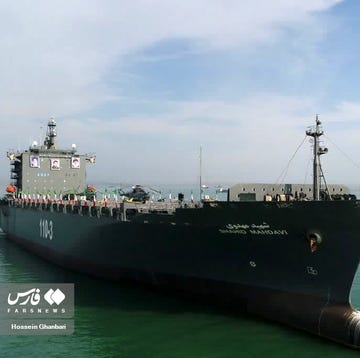
Q-Ships, the Weirdest Warships Ever, Are Back
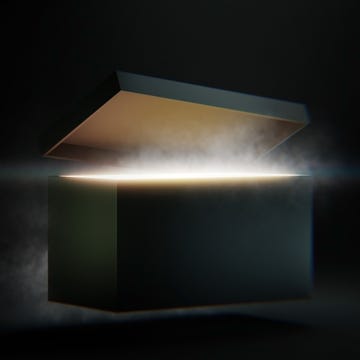
Archaeologists Dig Up 1,500-Year-Old Reliquary
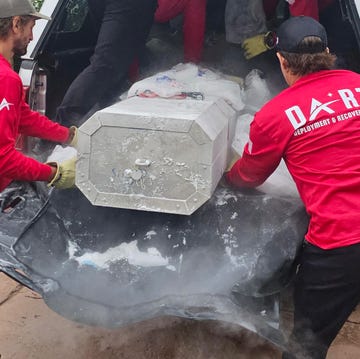
He Was Frozen in a Shed for 30 Years. Can He Rise?
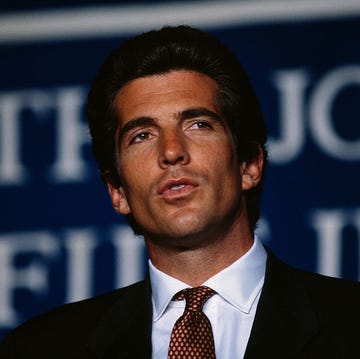
What Doomed JFK Jr.'s Final Flight?
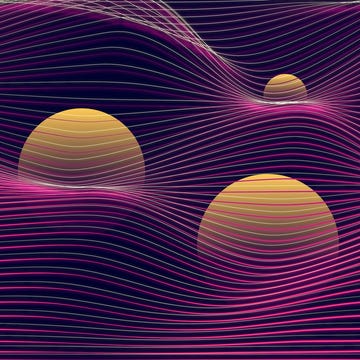
Why Everything We Know About Gravity May Be Wrong
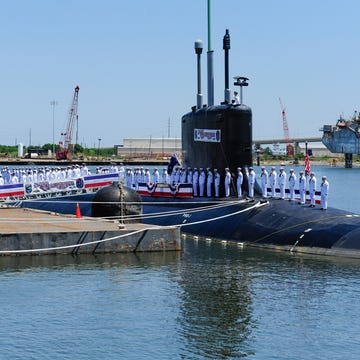
The Navy is Fixing its Ship-Naming Mess
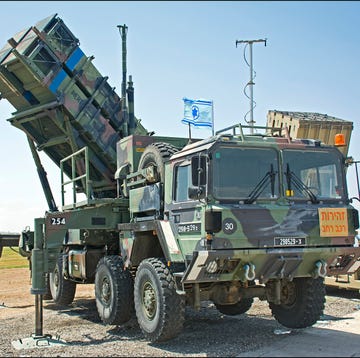
The U.S. May Buy Israeli Missiles for Ukraine
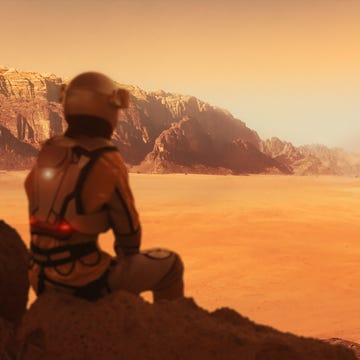
‘Stranded’ Astronauts Are Nothing New
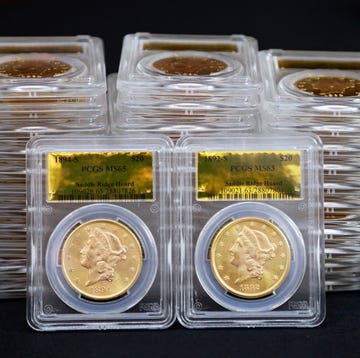
Could Buried Treasure Unlock a Civil War Mystery?
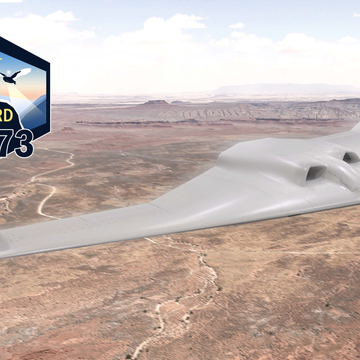
The Newest X-Plane is a Quiet, Stealthy Spy Plane
- The Speed of Light
Today anyone can use Google to search for the speed of light in a vaccuum and get an accurate result in seconds: 299 792 458 m/s . But who discovered the speed of light, and how did they do it?
Galileo Galilei was the first person to attempt to measure the speed of light, in the early 1600s. Galileo and an assistant each stood on a different hilltop with a known distance between them, the plan was for Galileo to open the shutter of a lamp and then for his assistant to open the shutter of a lamp as soon as he saw the light from Galileo's.
Using the distance between the hilltops and his pulse as a timer, Galileo planned to measure the speed of light. He and his assistant tried this with different distances between them, but no matter how far apart they were, he could measure no difference in the amount of time it took the light to travel.
Galileo concluded that the speed of light was too fast to be measured by this method, and he was correct. We now know the speed of light very precisely, and if Galileo and his assistant were on hilltops one mile apart, light would take 0.0000054 seconds to travel from one person to the other. It is understandable that Galileo was unable to measure this with his pulse!

Image credit: LCO
In 1676 a Danish astronomer named Ole Rømer was studying the orbits of the moons of Jupiter and making tables to predict when eclipses of the moons would occur. He noticed that when Jupiter and Earth are far apart (near conjunction ), the eclipses of the moons occurred several minutes later than when Jupiter and the Earth are closer (near opposition .) He reasoned that this could be because of the time light takes to travel from Jupiter to Earth.
Rømer found the maximum variation in timing of these eclipses to be 16.6 minutes. He interpreted this to be the amount of time it takes light to travel across the diameter of Earth's orbit. He didn't actually calculate the speed of light as the diameter of Earth's orbit was not well known in his day. But using his method with the knowledge of distances we have today, we get a value of approximately 301,204.8 km/s for the speed of light. This is only about 0.5% off the modern known value of the speed of light.

Léon Foucault
In the 1850s, French physicist Léon Foucault measured the speed of light in a laboratory using a light source, a rapidly rotating mirror and a stationary mirror. This method was based on a similar apparatus built by Armand-Hippolyte Fizeau. For the first time the speed of light could be measured on Earth, and the speed of light was measured to very great accuracy.
In the 1970s, interferometry was used to get the most accurate value for the speed of light that had been measured yet: 299,792.4562±0.0011 km/s. Then, in 1983, the meter was redefined in the International System of Units (SI) as the distance traveled by light in vacuum in 1/299,792,458 of a second. As a result, the numerical value of the speed of light ( c ) in meters per second is now fixed exactly by the definition of the meter. It is always slower in other materials such as water or glass. For most calculations the value 3.00 x 10 5 km/s is used.
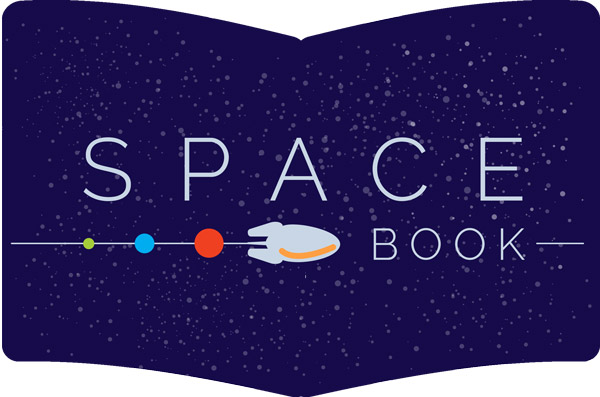
In this Chapter
- What is Light?
- Light as a Wave
- Light as a Particle
- Black-body Radiation
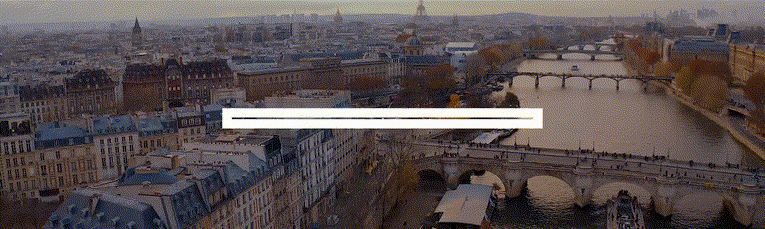
IMAGES
VIDEO
COMMENTS
The scientific breakthrough in electromagnetism by James Maxwell in 1860s shed light on a new way of measuring speed of light. Rosa and Dorsey used the electric permittivity ( ε) and magnetic permeability ( µ) constants to calculate the speed of light. c = 1 √εμ c = 1 ε μ. This method differed from historical methods in that it did not ...
However, if R rotates rapidly, the time delay due to the finite speed of light traveling from R to M and back to R results in the reflected image of the slit at S becoming displaced. 1850 experiment Figure 2: Foucault's determination of the relative speed of light in air vs water.
In fact, we now define the speed of light to be a constant, with a precise speed of 299,792,458 meters per second. While it remains a remote possibility in deeply theoretical physics that light ...
The experiment showed that it is possible for light to travel at a slower speed even in free space—and Padgett and his colleagues did it at the scale of individual photons.
The Michelson-Morley experiment was an attempt to measure the motion of the Earth relative to the luminiferous aether, a supposed medium permeating space that was thought to be the carrier of light waves.The experiment was performed between April and July 1887 by American physicists Albert A. Michelson and Edward W. Morley at what is now Case Western Reserve University in Cleveland, Ohio ...
Light - Michelson-Morley, Experiment, Wave-Particle: The German-born American physicist A.A. Michelson set the early standard for measurements of the speed of light in the late 1870s, determining a speed within 0.02 percent of the modern value. Michelson's most noteworthy measurements of the speed of light, however, were yet to come. From the first speculations on the wave nature of light by ...
In 1983, an international commission on weights and measures set the speed of light in a vacuum at the calculation we use today: 299,792,458 meters per second (186,282 miles per second)—a speed ...
2) Magnetic Explosions. 3) Wave-Particle Interactions. One hundred years ago today, on May 29, 1919, measurements of a solar eclipse offered verification for Einstein's theory of general relativity. Even before that, Einstein had developed the theory of special relativity, which revolutionized the way we understand light.
Foucault concluded in 1862 that the speed of light was 299,796 kilometers per second. Fizeau's contributions to science are not limited to this speed-of-light measurement. Subsequent experiments in which he measured how light traveled through flowing liquid resulted in a surprising discovery: the velocity of light doesn't change as expected ...
The speed of light in vacuum, commonly denoted c, is a universal physical constant that is exactly equal to 299,792,458 metres per second (approximately 300,000 kilometres per second; 186,000 miles per second; 671 million miles per hour). According to the special theory of relativity, c is the upper limit for the speed at which conventional matter or energy (and thus any signal carrying ...
Here's How. Scientists Believe Light Speed Travel Is Possible. Here's How. A functioning warp drive would allow humans to reach the far ends of the cosmos in the blink of an eye. n late 2020 ...
Speed of light experiments measure the average speed to a destination and back, leaving open the possibility that the speed may differ over each leg. Now Canadian physicists have measured the one ...
Physicists Have Broken The Speed of Light With Pulses Inside Hot Plasma. Sailing through the smooth waters of vacuum, a photon of light moves at around 300 thousand kilometers (186 thousand miles) a second. This sets a firm limit on how quickly a whisper of information can travel anywhere in the Universe. While this law isn't likely to ever be ...
the experiment. 2 Theory 2.1 Speed of light The following scenario is about two light sources inserted in a tube that is evacuated. One ... This implies light will take the same duration to travel a distance 2 x+ 2l m in air as to travel a distance of 2l m in a medium. With that in mind and the
It matches the speed of a gravitational wave, and yes, it's the same c that's in the famous equation E=mc 2. We don't just have the word of Maxwell and Einstein for what the speed of light is, though. Scientists have measured it by bouncing lasers back from objects and watching the way gravity acts on planets, and all these experiments come up ...
1 To travel backward in time, the spacecraft's velocity must exceed: where u is the velocity of the planet relative to Earth, and c is the speed of light. Seth Lloyd, professor of quantum ...
The trouble was that the answer didn't make sense. The first tentative calculation of tunneling time appeared in print in 1932. Even earlier stabs might have been made in private, but "when you get an answer you can't make sense of, you don't publish it," noted Aephraim Steinberg, a physicist at the University of Toronto.. It wasn't until 1962 that a semiconductor engineer at Texas ...
The free STEM projects, experiments, lessons and activities below help educators teach K-12 students about the physics of light, specifically, visible light, with hands-on exploration and active learning. The resources below have been grouped by grade band to help educators select the experiments and lessons that best fit their needs.
When using the term "the speed of light" it is sometimes necessary to make the distinction between its one-way speed and its two-way speed.The "one-way" speed of light, from a source to a detector, cannot be measured independently of a convention as to how to synchronize the clocks at the source and the detector.What can however be experimentally measured is the round-trip speed (or "two-way ...
From his thought experiment and observations, Bradley concluded that the speed of light is around 297,729 kilometers per second. This only about 1 % of the mark!! Pretty incredible.
In 1676, the Danish astronomer Ole Roemer (1644-1710) became the first person to measure the speed of light. Roemer measured the speed of light by timing eclipses of Jupiter's moon Io. In this figure, S is the Sun, E1 is the Earth when closest to Jupiter (J1) and E2 is the Earth about six months later, on the opposite side of the Sun from ...
This method was based on a similar apparatus built by Armand-Hippolyte Fizeau. For the first time the speed of light could be measured on Earth, and the speed of light was measured to very great accuracy. In the 1970s, interferometry was used to get the most accurate value for the speed of light that had been measured yet: 299,792.4562±0.0011 ...
Learn about the finite speed of light with this video segment adapted from Shedding Light on Science. In the 1600s, Galileo investigated the speed of light by conducting an experiment with lanterns, but he was unsuccessful in determining whether light had a measurable speed. It wasn't until later that Danish astronomer Ole Roemer calculated a finite speed of light by studying Jupiter's moon, Io.