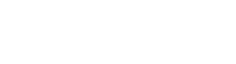
- Login/Register
- Solar System
- Exotic Objects
- Upcoming Events
- Deep-Sky Objects
- Observing Basics
- Telescopes and Equipment
- Astrophotography
- Space Exploration
- Human Spaceflight
- Robotic Spaceflight
- The Magazine
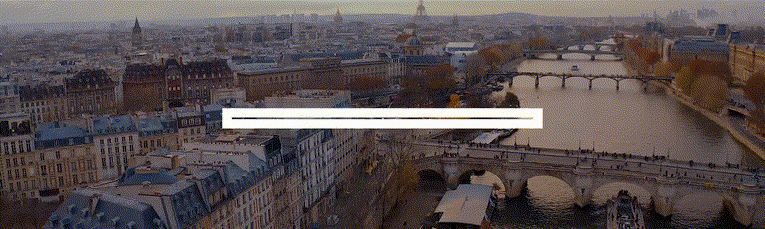
What is the speed of light? Here’s the history, discovery of the cosmic speed limit
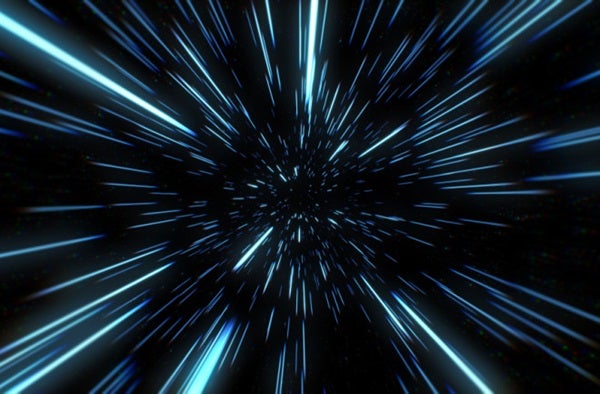
On one hand, the speed of light is just a number: 299,792,458 meters per second. And on the other, it’s one of the most important constants that appears in nature and defines the relationship of causality itself.
As far as we can measure, it is a constant. It is the same speed for every observer in the entire universe. This constancy was first established in the late 1800’s with the experiments of Albert Michelson and Edward Morley at Case Western Reserve University . They attempted to measure changes in the speed of light as the Earth orbited around the Sun. They found no such variation, and no experiment ever since then has either.
Observations of the cosmic microwave background, the light released when the universe was 380,000 years old, show that the speed of light hasn’t measurably changed in over 13.8 billion years.
In fact, we now define the speed of light to be a constant, with a precise speed of 299,792,458 meters per second. While it remains a remote possibility in deeply theoretical physics that light may not be a constant, for all known purposes it is a constant, so it’s better to just define it and move on with life.
How was the speed of light first measured?
In 1676 the Danish astronomer Ole Christensen Romer made the first quantitative measurement of how fast light travels. He carefully observed the orbit of Io, the innermost moon of Jupiter. As the Earth circles the Sun in its own orbit, sometimes it approaches Jupiter and sometimes it recedes away from it. When the Earth is approaching Jupiter, the path that light has to travel from Io is shorter than when the Earth is receding away from Jupiter. By carefully measuring the changes to Io’s orbital period, Romer calculated a speed of light of around 220,000 kilometers per second.
Observations continued to improve until by the 19 th century astronomers and physicists had developed the sophistication to get very close to the modern value. In 1865, James Clerk Maxwell made a remarkable discovery. He was investigating the properties of electricity and magnetism, which for decades had remained mysterious in unconnected laboratory experiments around the world. Maxwell found that electricity and magnetism were really two sides of the same coin, both manifestations of a single electromagnetic force.
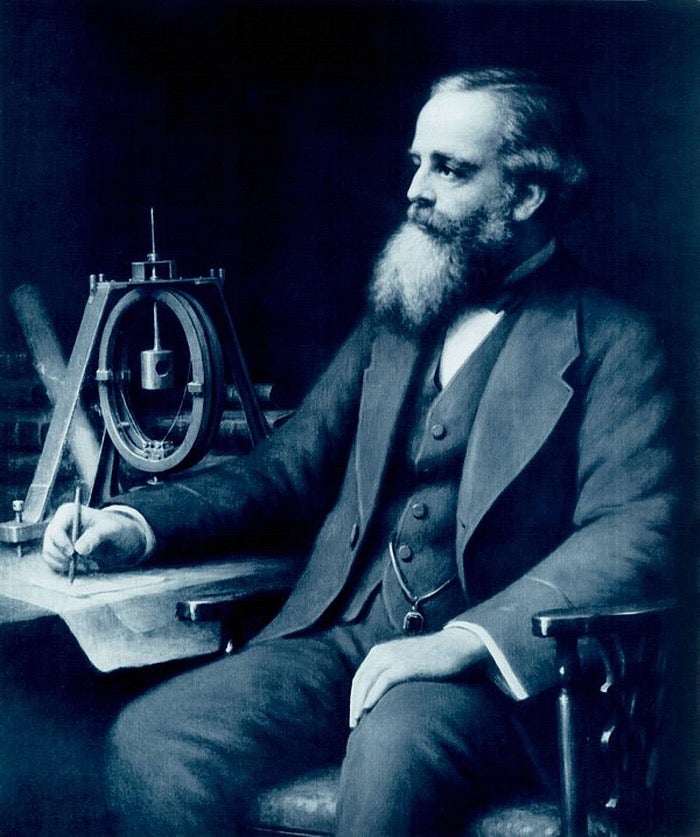
As Maxwell explored the consequences of his new theory, he found that changing magnetic fields can lead to changing electric fields, which then lead to a new round of changing magnetic fields. The fields leapfrog over each other and can even travel through empty space. When Maxwell went to calculate the speed of these electromagnetic waves, he was surprised to see the speed of light pop out – the first theoretical calculation of this important number.
What is the most precise measurement of the speed of light?
Because it is defined to be a constant, there’s no need to measure it further. The number we’ve defined is it, with no uncertainty, no error bars. It’s done. But the speed of light is just that – a speed. The number we choose to represent it depends on the units we use: kilometers versus miles, seconds versus hours, and so on. In fact, physicists commonly just set the speed of light to be 1 to make their calculations easier. So instead of trying to measure the speed light travels, physicists turn to more precisely measuring other units, like the length of the meter or the duration of the second. In other words, the defined value of the speed of light is used to establish the length of other units like the meter.
How does light slow down?
Yes, the speed of light is always a constant. But it slows down whenever it travels through a medium like air or water. How does this work? There are a few different ways to present an answer to this question, depending on whether you prefer a particle-like picture or a wave-like picture.
In a particle-like picture, light is made of tiny little bullets called photons. All those photons always travel at the speed of light, but as light passes through a medium those photons get all tangled up, bouncing around among all the molecules of the medium. This slows down the overall propagation of light, because it takes more time for the group of photons to make it through.
In a wave-like picture, light is made of electromagnetic waves. When these waves pass through a medium, they get all the charged particles in motion, which in turn generate new electromagnetic waves of their own. These interfere with the original light, forcing it to slow down as it passes through.
Either way, light always travels at the same speed, but matter can interfere with its travel, making it slow down.
Why is the speed of light important?
The speed of light is important because it’s about way more than, well, the speed of light. In the early 1900’s Einstein realized just how special this speed is. The old physics, dominated by the work of Isaac Newton, said that the universe had a fixed reference frame from which we could measure all motion. This is why Michelson and Morley went looking for changes in the speed, because it should change depending on our point of view. But their experiments showed that the speed was always constant, so what gives?
Einstein decided to take this experiment at face value. He assumed that the speed of light is a true, fundamental constant. No matter where you are, no matter how fast you’re moving, you’ll always see the same speed.
This is wild to think about. If you’re traveling at 99% the speed of light and turn on a flashlight, the beam will race ahead of you at…exactly the speed of light, no more, no less. If you’re coming from the opposite direction, you’ll still also measure the exact same speed.
This constancy forms the basis of Einstein’s special theory of relativity, which tells us that while all motion is relative – different observers won’t always agree on the length of measurements or the duration of events – some things are truly universal, like the speed of light.
Can you go faster than light speed?
Nope. Nothing can. Any particle with zero mass must travel at light speed. But anything with mass (which is most of the universe) cannot. The problem is relativity. The faster you go, the more energy you have. But we know from Einstein’s relativity that energy and mass are the same thing. So the more energy you have, the more mass you have, which makes it harder for you to go even faster. You can get as close as you want to the speed of light, but to actually crack that barrier takes an infinite amount of energy. So don’t even try.
How is the speed at which light travels related to causality?
If you think you can find a cheat to get around the limitations of light speed, then I need to tell you about its role in special relativity. You see, it’s not just about light. It just so happens that light travels at this special speed, and it was the first thing we discovered to travel at this speed. So it could have had another name. Indeed, a better name for this speed might be “the speed of time.”
Related: Is time travel possible? An astrophysicist explains
We live in a universe of causes and effects. All effects are preceded by a cause, and all causes lead to effects. The speed of light limits how quickly causes can lead to effects. Because it’s a maximum speed limit for any motion or interaction, in a given amount of time there’s a limit to what I can influence. If I want to tap you on the shoulder and you’re right next to me, I can do it right away. But if you’re on the other side of the planet, I have to travel there first. The motion of me traveling to you is limited by the speed of light, so that sets how quickly I can tap you on the shoulder – the speed light travels dictates how quickly a single cause can create an effect.
The ability to go faster than light would allow effects to happen before their causes. In essence, time travel into the past would be possible with faster-than-light travel. Since we view time as the unbroken chain of causes and effects going from the past to the future, breaking the speed of light would break causality, which would seriously undermine our sense of the forward motion of time.
Why does light travel at this speed?
No clue. It appears to us as a fundamental constant of nature. We have no theory of physics that explains its existence or why it has the value that it does. We hope that a future understanding of nature will provide this explanation, but right now all investigations are purely theoretical. For now, we just have to take it as a given.
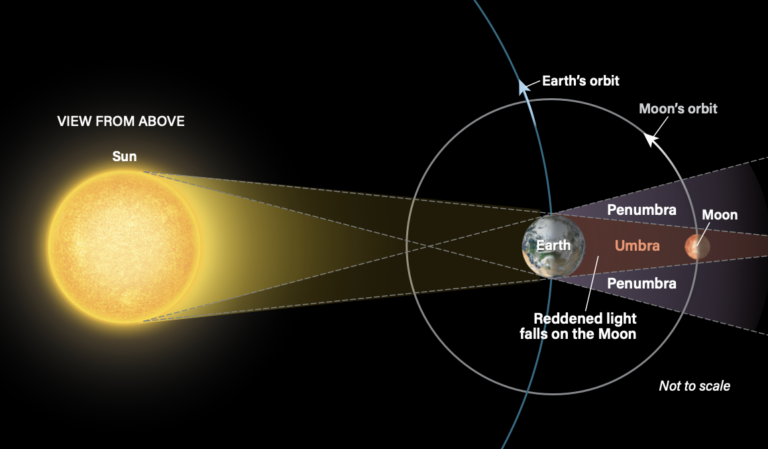
Why can we still see the Moon’s disk during a total lunar eclipse?
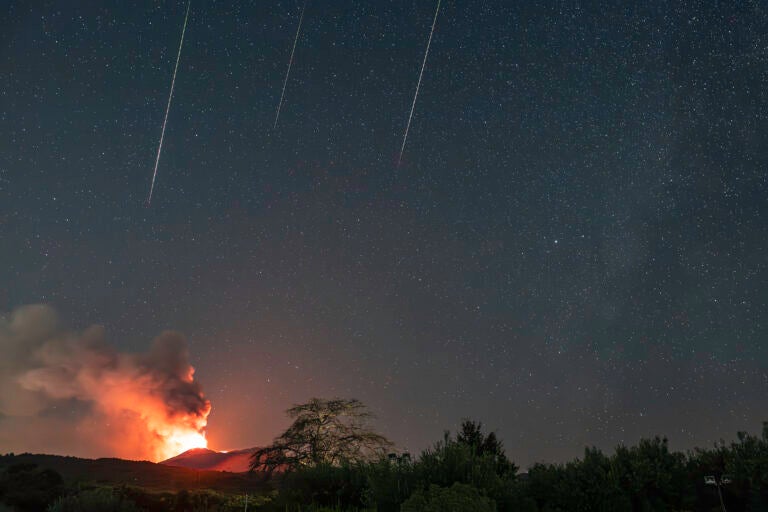
Could we experience the first-ever human-made meteor shower?
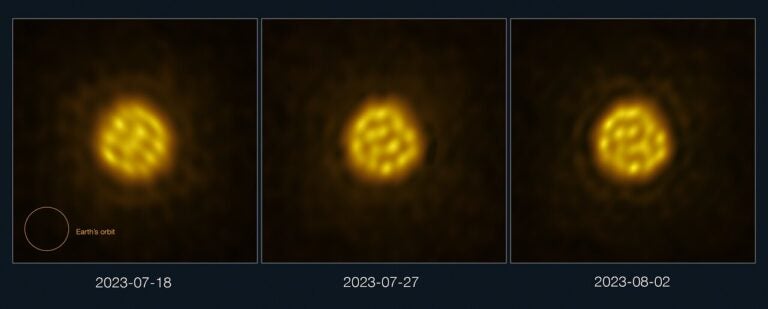
ALMA reveals a star’s surface in unprecedented detail
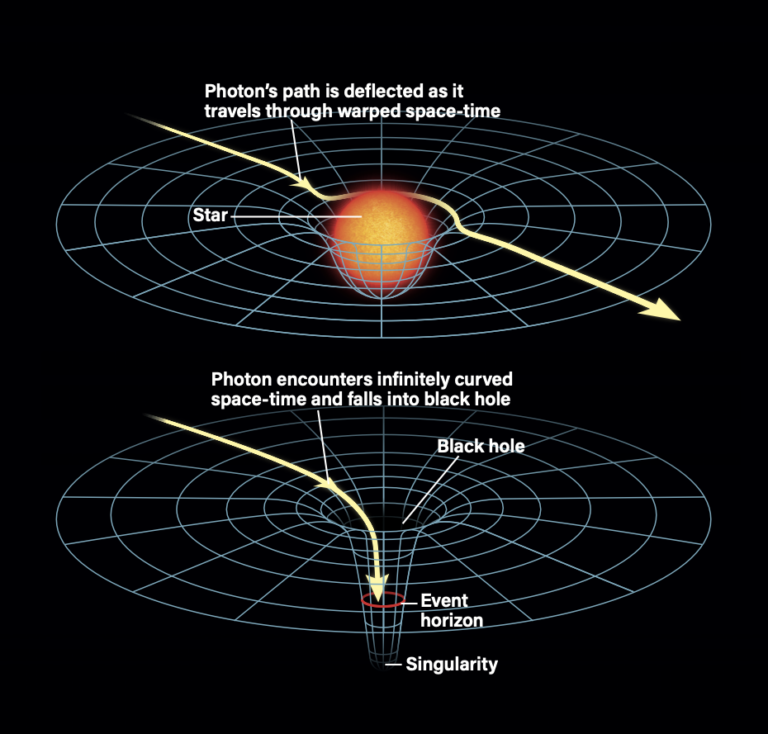
How can a black hole pull light into itself if a photon is massless?
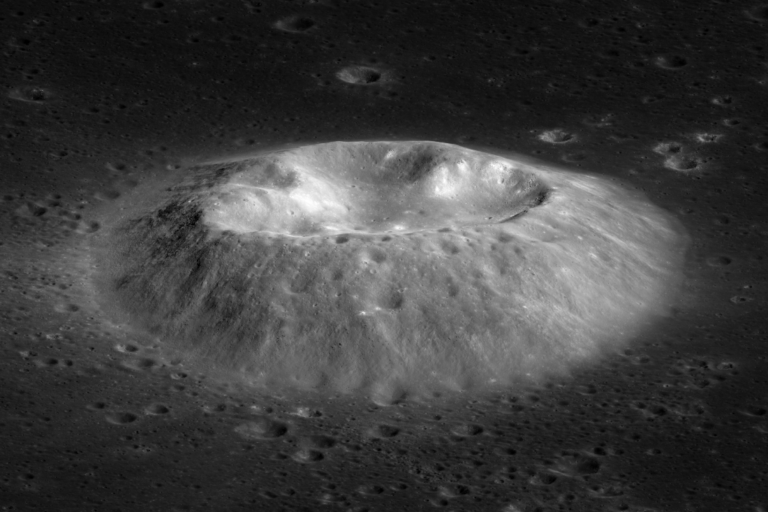
Volcanoes were erupting on the Moon while dinosaurs roamed Earth, Chang’e 5 samples suggest
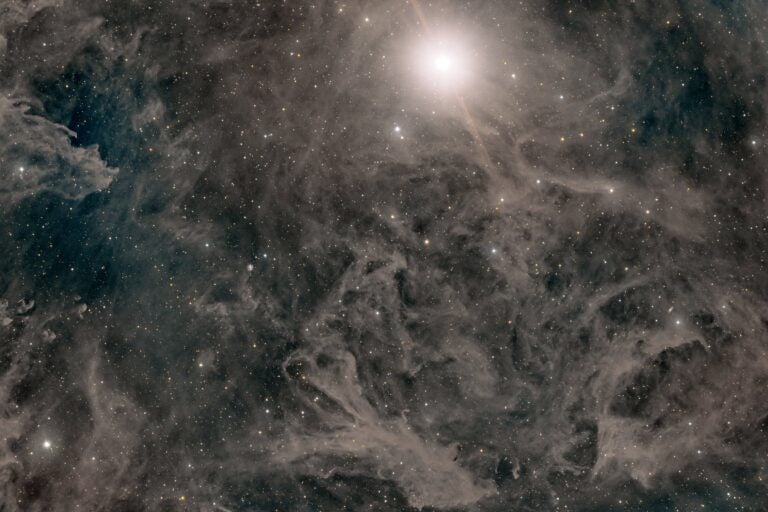
New Horizons measures how dark the universe can get
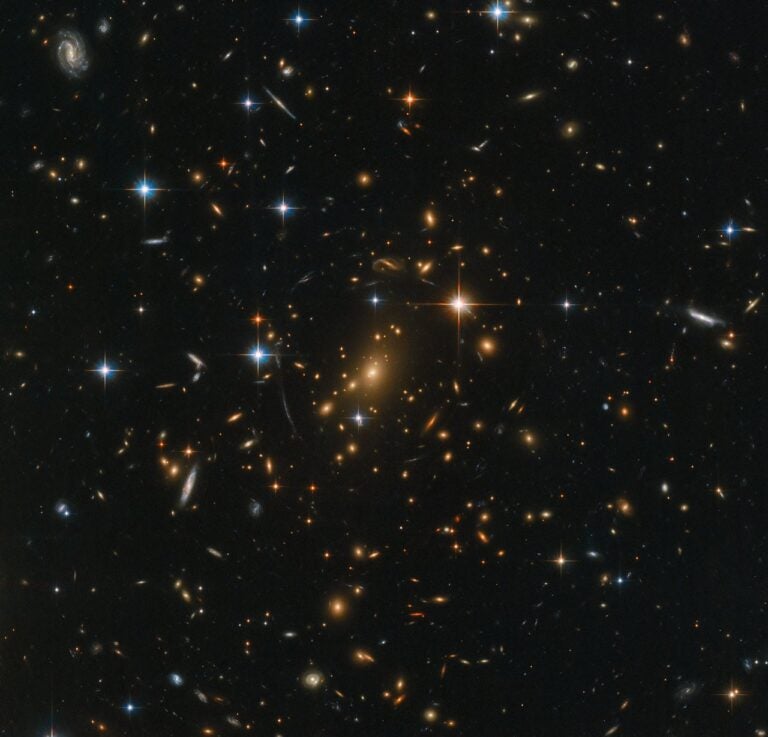
What we do — and don’t — know about dark energy
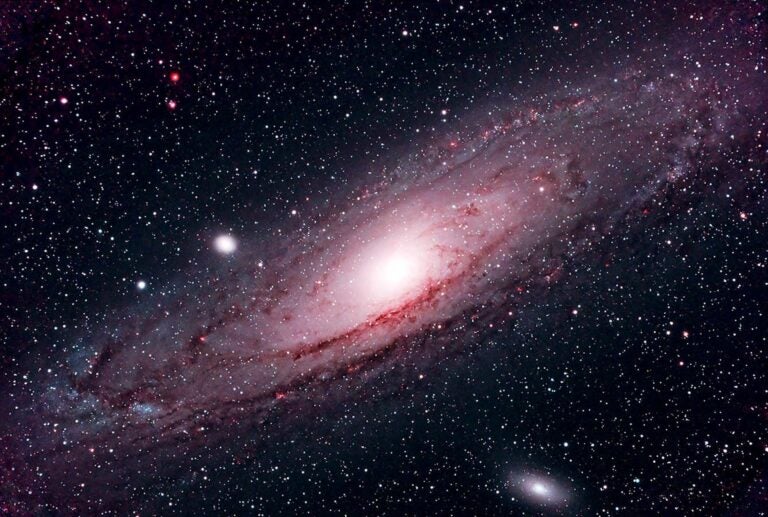
Get to know the Andromeda Galaxy
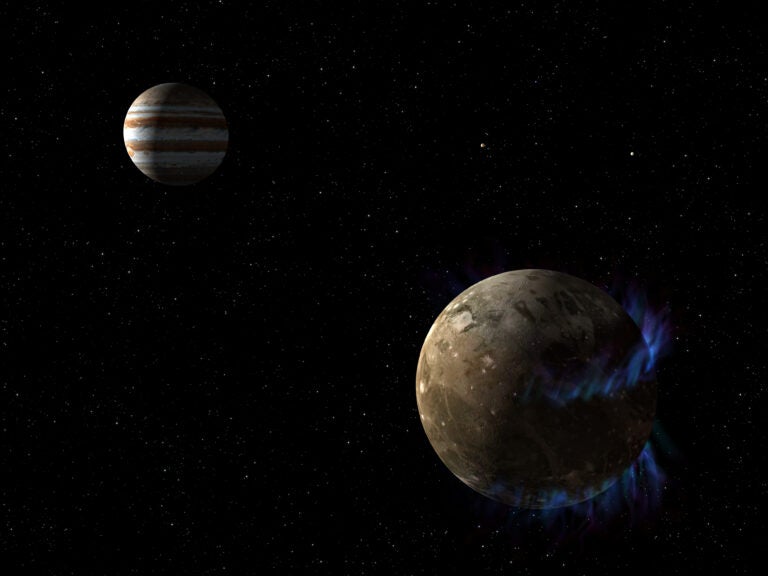
Scientists view Ganymede’s aurorae dancing during eclipse
Speed of Light Calculator
Table of contents
With this speed of light calculator, we aim to help you calculate the distance light can travel in a fixed time . As the speed of light is the fastest speed in the universe, it would be fascinating to know just how far it can travel in a short amount of time.
We have written this article to help you understand what the speed of light is , how fast the speed of light is , and how to calculate the speed of light . We will also demonstrate some examples to help you understand the computation of the speed of light.
What is the speed of light? How fast is the speed of light?
The speed of light is scientifically proven to be the universe's maximum speed. This means no matter how hard you try, you can never exceed this speed in this universe. Hence, there are also some theories on getting into another universe by breaking this limit. You can understand this more using our speed calculator and distance calculator .
So, how fast is the speed of light? The speed of light is 299,792,458 m/s in a vacuum. The speed of light in mph is 670,616,629 mph . With this speed, one can go around the globe more than 400,000 times in a minute!
One thing to note is that the speed of light slows down when it goes through different mediums. Light travels faster in air than in water, for instance. This phenomenon causes the refraction of light.
Now, let's look at how to calculate the speed of light.
How to calculate the speed of light?
As the speed of light is constant, calculating the speed of light usually falls on calculating the distance that light can travel in a certain time period. Hence, let's have a look at the following example:
- Source: Light
- Speed of light: 299,792,458 m/s
- Time traveled: 100 seconds
You can perform the calculation in three steps:
Determine the speed of light.
As mentioned, the speed of light is the fastest speed in the universe, and it is always a constant in a vacuum. Hence, the speed of light is 299,792,458 m/s .
Determine the time that the light has traveled.
The next step is to know how much time the light has traveled. Unlike looking at the speed of a sports car or a train, the speed of light is extremely fast, so the time interval that we look at is usually measured in seconds instead of minutes and hours. You can use our time lapse calculator to help you with this calculation.
For this example, the time that the light has traveled is 100 seconds .
Calculate the distance that the light has traveled.
The final step is to calculate the total distance that the light has traveled within the time . You can calculate this answer using the speed of light formula:
distance = speed of light × time
Thus, the distance that the light can travel in 100 seconds is 299,792,458 m/s × 100 seconds = 29,979,245,800 m
What is the speed of light in mph when it is in a vacuum?
The speed of light in a vacuum is 670,616,629 mph . This is equivalent to 299,792,458 m/s or 1,079,252,849 km/h. This is the fastest speed in the universe.
Is the speed of light always constant?
Yes , the speed of light is always constant for a given medium. The speed of light changes when going through different mediums. For example, light travels slower in water than in air.
How can I calculate the speed of light?
You can calculate the speed of light in three steps:
Determine the distance the light has traveled.
Apply the speed of light formula :
speed of light = distance / time
How far can the speed of light travel in 1 minute?
Light can travel 17,987,547,480 m in 1 minute . This means that light can travel around the earth more than 448 times in a minute.
Speed of light
The speed of light in the medium. In a vacuum, the speed of light is 299,792,458 m/s.

Why is the speed of light the way it is?
It's just plain weird.
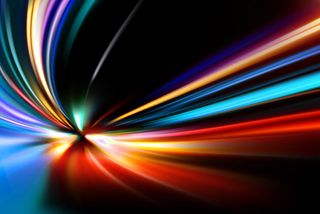
Paul M. Sutter is an astrophysicist at SUNY Stony Brook and the Flatiron Institute, host of Ask a Spaceman and Space Radio , and author of " How to Die in Space ." He contributed this article to Space.com's Expert Voices: Op-Ed & Insights .
We all know and love the speed of light — 299,792,458 meters per second — but why does it have the value that it does? Why isn't it some other number? And why do we care so much about some random speed of electromagnetic waves? Why did it become such a cornerstone of physics?
Well, it's because the speed of light is just plain weird.
Related: Constant speed of light: Einstein's special relativity survives a high-energy test
Putting light to the test
The first person to realize that light does indeed have a speed at all was an astronomer by the name of Ole Romer. In the late 1600s, he was obsessed with some strange motions of the moon Io around Jupiter. Every once in a while, the great planet would block our view of its little moon, causing an eclipse, but the timing between eclipses seemed to change over the course of the year. Either something funky was happening with the orbit of Io — which seemed suspicious — or something else was afoot.
After a couple years of observations, Romer made the connection. When we see Io get eclipsed, we're in a certain position in our own orbit around the sun. But by the next time we see another eclipse, a few days later, we're in a slightly different position, maybe closer or farther away from Jupiter than the last time. If we are farther away than the last time we saw an eclipse, then that means we have to wait a little bit of extra time to see the next one because it takes that much longer for the light to reach us, and the reverse is true if we happen to be a little bit closer to Jupiter.
The only way to explain the variations in the timing of eclipses of Io is if light has a finite speed.
Get the Space.com Newsletter
Breaking space news, the latest updates on rocket launches, skywatching events and more!
Making it mean something
Continued measurements over the course of the next few centuries solidified the measurement of the speed of light, but it wasn't until the mid-1800s when things really started to come together. That's when the physicist James Clerk Maxwell accidentally invented light.
Maxwell had been playing around with the then-poorly-understood phenomena of electricity and magnetism when he discovered a single unified picture that could explain all the disparate observations. Laying the groundwork for what we now understand to be the electromagnetic force , in those equations he discovered that changing electric fields can create magnetic fields, and vice versa. This allows waves of electricity to create waves of magnetism, which go on to make waves of electricity and back and forth and back and forth, leapfrogging over each other, capable of traveling through space.
And when he went to calculate the speed of these so-called electromagnetic waves, Maxwell got the same number that scientists had been measuring as the speed of light for centuries. Ergo, light is made of electromagnetic waves and it travels at that speed, because that is exactly how quickly waves of electricity and magnetism travel through space.
And this was all well and good until Einstein came along a few decades later and realized that the speed of light had nothing to do with light at all. With his special theory of relativity , Einstein realized the true connection between time and space, a unified fabric known as space-time. But as we all know, space is very different than time. A meter or a foot is very different than a second or a year. They appear to be two completely different things.
So how could they possibly be on the same footing?
There needed to be some sort of glue, some connection that allowed us to translate between movement in space and movement in time. In other words, we need to know how much one meter of space, for example, is worth in time. What's the exchange rate? Einstein found that there was a single constant, a certain speed, that could tell us how much space was equivalent to how much time, and vice versa.
Einstein's theories didn't say what that number was, but then he applied special relativity to the old equations of Maxwell and found that this conversion rate is exactly the speed of light.
Of course, this conversion rate, this fundamental constant that unifies space and time, doesn't know what an electromagnetic wave is, and it doesn't even really care. It's just some number, but it turns out that Maxwell had already calculated this number and discovered it without even knowing it. That's because all massless particles are able to travel at this speed, and since light is massless, it can travel at that speed. And so, the speed of light became an important cornerstone of modern physics.
But still, why that number, with that value, and not some other random number? Why did nature pick that one and no other? What's going on?
Related: The genius of Albert Einstein: his life, theories and impact on science
Making it meaningless
Well, the number doesn't really matter. It has units after all: meters per second. And in physics any number that has units attached to it can have any old value it wants, because it means you have to define what the units are. For example, in order to express the speed of light in meters per second, first you need to decide what the heck a meter is and what the heck a second is. And so the definition of the speed of light is tied up with the definitions of length and time.
In physics, we're more concerned with constants that have no units or dimensions — in other words, constants that appear in our physical theories that are just plain numbers. These appear much more fundamental, because they don't depend on any other definition. Another way of saying it is that, if we were to meet some alien civilization , we would have no way of understanding their measurement of the speed of light, but when it comes to dimensionless constants, we can all agree. They're just numbers.
One such number is known as the fine structure constant, which is a combination of the speed of light, Planck's constant , and something known as the permittivity of free space. Its value is approximately 0.007. 0.007 what? Just 0.007. Like I said, it's just a number.
So on one hand, the speed of light can be whatever it wants to be, because it has units and we need to define the units. But on the other hand, the speed of light can't be anything other than exactly what it is, because if you were to change the speed of light, you would change the fine structure constant. But our universe has chosen the fine structure constant to be approximately 0.007, and nothing else. That is simply the universe we live in, and we get no choice about it at all. And since this is fixed and universal, the speed of light has to be exactly what it is.
So why is the fine structure constant exactly the number that it is, and not something else? Good question. We don't know.
Learn more by listening to the episode "Why is the speed of light the way it is?" on the Ask A Spaceman podcast, available on iTunes and on the Web at http://www.askaspaceman.com. Thanks to Robert H, Michael E., @DesRon94, Evan W., Harry A., @twdixon, Hein P., Colin E., and Lothian53 for the questions that led to this piece! Ask your own question on Twitter using #AskASpaceman or by following Paul @PaulMattSutter and facebook.com/PaulMattSutter.
Join our Space Forums to keep talking space on the latest missions, night sky and more! And if you have a news tip, correction or comment, let us know at: [email protected].
Paul M. Sutter is an astrophysicist at SUNY Stony Brook and the Flatiron Institute in New York City. Paul received his PhD in Physics from the University of Illinois at Urbana-Champaign in 2011, and spent three years at the Paris Institute of Astrophysics, followed by a research fellowship in Trieste, Italy, His research focuses on many diverse topics, from the emptiest regions of the universe to the earliest moments of the Big Bang to the hunt for the first stars. As an "Agent to the Stars," Paul has passionately engaged the public in science outreach for several years. He is the host of the popular "Ask a Spaceman!" podcast, author of "Your Place in the Universe" and "How to Die in Space" and he frequently appears on TV — including on The Weather Channel, for which he serves as Official Space Specialist.
The bubbling surface of a distant star was captured on video for the 1st time ever
Just how dark is the universe? NASA's New Horizons probe gives us best estimate yet
Could we turn the sun into a gigantic telescope?
- voidpotentialenergy This is just my opinion but i think L speed is it's speed because the particle part of it is the fastest it can interact with the quanta distance in quantum fluctuation. Light is particle and wave so the wave happens in the void between quanta. Gravity probably travels in that void and why gravity seems instant. Reply
- rod The space.com article wraps up the discussion with, "So on one hand, the speed of light can be whatever it wants to be, because it has units and we need to define the units. But on the other hand, the speed of light can't be anything other than exactly what it is, because if you were to change the speed of light, you would change the fine structure constant. But our universe has chosen the fine structure constant to be approximately 0.007, and nothing else. That is simply the universe we live in, and we get no choice about it at all. And since this is fixed and universal, the speed of light has to be exactly what it is. So why is the fine structure constant exactly the number that it is, and not something else? Good question. We don't know." It seems that the *universe* made this decision, *But our universe has chosen the fine structure constant to be...* I did not know that the universe was capable of making decisions concerning constants used in physics. E=mc^2 is a serious constant. Look at nuclear weapons development, explosive yields, and stellar evolution burn rates for p-p chain and CNO fusion rates. The report indicates why alpha (fine structure constant) is what it is and c is what it is, *We don't know*. Reply
Admin said: We all know and love the speed of light, but why does it have the value that it does? Why isn't it some other number? And why did it become such a cornerstone of physics? Why is the speed of light the way it is? : Read more
rod said: The space.com article wraps up the discussion with, "So on one hand, the speed of light can be whatever it wants to be, because it has units and we need to define the units. But on the other hand, the speed of light can't be anything other than exactly what it is, because if you were to change the speed of light, you would change the fine structure constant. But our universe has chosen the fine structure constant to be approximately 0.007, and nothing else. That is simply the universe we live in, and we get no choice about it at all. And since this is fixed and universal, the speed of light has to be exactly what it is. So why is the fine structure constant exactly the number that it is, and not something else? Good question. We don't know." It seems that the *universe* made this decision, *But our universe has chosen the fine structure constant to be...* I did not know that the universe was capable of making decisions concerning constants used in physics. E=mc^2 is a serious constant. Look at nuclear weapons development, explosive yields, and stellar evolution burn rates for p-p chain and CNO fusion rates. The report indicates why alpha (fine structure constant) is what it is and c is what it is, *We don't know*.
- rod FYI. When someone says *the universe has chosen*, I am reminded of these five lessons from a 1982 Fed. court trial. The essential characteristics of science are: It is guided by natural law; It has to be explanatory by reference to natural law; It is testable against the empirical world; Its conclusions are tentative, i.e., are not necessarily the final word; and It is falsifiable. Five important points about science. Reply
- Gary If the universe is expanding , how can the speed of light be constant ( miles per second , if each mile is getting longer ) ? Can light's velocity be constant while the universe expands ? So, with the expansion of the universe , doesn't the speed of light need to increase in order to stay at a constant velocity in miles per second ? Or, do the miles in the universe remain the same length as the universe 'adds' miles to its diameter ? Are the miles lengthening or are they simply being added / compounded ? Reply
- Gary Lets say we're in outer space and we shoot a laser through a block of glass. What causes the speed of the laser light to return to the speed it held prior to entering the block of glass ? Is there some medium in the vacuum of space that governs the speed of light ? Do the atoms in the glass push it back up to its original speed. If so, why don't those same atoms constantly push the light while it travels through the block of glass ? Reply
Gary said: Lets say we're in outer space and we shoot a laser through a block of glass. What causes the speed of the laser light to return to the speed it held prior to entering the block of glass ? Is there some medium in the vacuum of space that governs the speed of light ? Do the atoms in the glass push it back up to its original speed. If so, why don't those same atoms constantly push the light while it travels through the block of glass ?
Gary said: If the universe is expanding , how can the speed of light be constant ( miles per second , if each mile is getting longer ) ? Can light's velocity be constant while the universe expands ? So, with the expansion of the universe , doesn't the speed of light need to increase in order to stay at a constant velocity in miles per second ? Or, do the miles in the universe remain the same length as the universe 'adds' miles to its diameter ? Are the miles lengthening or are they simply being added / compounded ?
- View All 31 Comments
Most Popular
- 2 SpaceX's private Polaris Dawn astronauts splash down to end historic spacewalk mission (video)
- 3 SpaceX's private Polaris Dawn astronauts will return to Earth early Sunday. Here's how to watch live online
- 4 Astronauts 3D-print 1st metal part while on ISS
- 5 This Week In Space podcast: Episode 128 — Starliner is Back! What Now?
What is the speed of light?
Light is faster than anything else in the known universe, though its speed can change depending on what it's passing through.
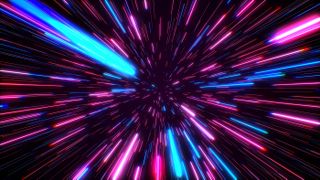
The universe has a speed limit, and it's the speed of light. Nothing can travel faster than light — not even our best spacecraft — according to the laws of physics.
So, what is the speed of light?
Light moves at an incredible 186,000 miles per second (300,000 kilometers per second), equivalent to almost 700 million mph (more than 1 billion km/h). That's fast enough to circumnavigate the globe 7.5 times in one second, while a typical passenger jet would take more than two days to go around once (and that doesn't include stops for fuel or layovers!).
Light moves so fast that, for much of human history, we thought it traveled instantaneously. As early as the late 1600s, though, scientist Ole Roemer was able to measure the speed of light (usually referred to as c ) by using observations of Jupiter's moons, according to Britannica .
Around the turn of the 19th century, physicist James Clerk Maxwell created his theories of electromagnetism . Light is itself made up of electric and magnetic fields, so electromagnetism could describe the behavior and motion of light — including its theoretical speed. That value was 299,788 kilometers per second, with a margin of error of plus or minus 30. In the 1970s, physicists used lasers to measure the speed of light with much greater precision, leaving an error of only 0.001. Nowadays, the speed of light is used to define units of length, so its value is fixed; humans have essentially agreed the speed of light is 299,792.458 kilometers per second, exactly.
Light doesn't always have to go so fast, though. Depending on what it's traveling through — air, water, diamonds, etc. — it can slow down. The official speed of light is measured as if it's traveling in a vacuum, a space with no air or anything to get in the way. You can most clearly see differences in the speed of light in something like a prism, where certain energies of light bend more than others, creating a rainbow.
— How many moons does Earth have ?
— What would happen if the moon were twice as close to Earth?
— If you're on the moon, does the Earth appear to go through phases?
Interestingly, the speed of light is no match for the vast distances of space, which is itself a vacuum. It takes 8 minutes for light from the sun to reach Earth, and a couple years for light from the other closest stars (like Proxima Centauri) to get to our planet. This is why astronomers use the unit light-years — the distance light can travel in one year — to measure vast distances in space.
Sign up for the Live Science daily newsletter now
Get the world’s most fascinating discoveries delivered straight to your inbox.
Because of this universal speed limit, telescopes are essentially time machines . When astronomers look at a star 500 light-years away, they're looking at light from 500 years ago. Light from around 13 billion light-years away (equivalently, 13 billion years ago) shows up as the cosmic microwave background, remnant radiation from the Big Bang in the universe's infancy. The speed of light isn't just a quirk of physics; it has enabled modern astronomy as we know it, and it shapes the way we see the world — literally.
Briley Lewis (she/her) is a freelance science writer and Ph.D. Candidate/NSF Fellow at the University of California, Los Angeles studying Astronomy & Astrophysics. Follow her on Twitter @briles_34 or visit her website www.briley-lewis.com .
One of the universe's biggest paradoxes could be even weirder than we thought, James Webb telescope study reveals
Early galaxies weren't mystifyingly massive after all, James Webb Space Telescope finds
Color-blind people may be less picky eaters. Here's why.
Most Popular
- 2 Angular roughshark: The pig-faced shark that grunts when captured
- 3 The moon might still have active volcanoes, China's Chang'e 5 sample-return probe reveals
- 4 How did people clean themselves before soap was invented?
- 5 'I have never written of a stranger organ': The rise of the placenta and how it helped make us human
Learn About the True Speed of Light and How It's Used
Roberto Moiola/Sysaworld/Getty Images
- An Introduction to Astronomy
- Important Astronomers
- Solar System
- Stars, Planets, and Galaxies
- Space Exploration
- Weather & Climate
- Ph.D., Physics and Astronomy, Purdue University
- B.S., Physics, Purdue University
Light moves through the universe at the fastest speed astronomers can measure. In fact, the speed of light is a cosmic speed limit, and nothing is known to move faster. How fast does light move? This limit can be measured and it also helps define our understanding of the universe's size and age.
What Is Light: Wave or Particle?
Light travels fast, at a velocity of 299, 792, 458 meters per second. How can it do this? To understand that, it's helpful to know what light actually is and that's largely a 20th-century discovery.
The nature of light was a great mystery for centuries. Scientists had trouble grasping the concept of its wave and particle nature. If it was a wave what did it propagate through? Why did it appear to travel at the same speed in all directions? And, what can the speed of light tell us about the cosmos? It wasn't until Albert Einstein described this theory of special relativity in 1905 it all came into focus. Einstein argued that space and time were relative and that the speed of light was the constant that connected the two.
What Is the Speed of Light?
It is often stated that the speed of light is constant and that nothing can travel faster than the speed of light. This isn't entirely accurate. The value of 299,792,458 meters per second (186,282 miles per second) is the speed of light in a vacuum. However, light actually slows down as it passes through different media. For instance, when it moves through glass, it slows down to about two-thirds of its speed in a vacuum. Even in air, which is nearly a vacuum, light slows down slightly. As it moves through space, it encounters clouds of gas and dust, as well as gravitational fields, and those can change the speed a tiny bit. The clouds of gas and dust also absorb some of the light as it passes through.
This phenomenon has to do with the nature of light, which is an electromagnetic wave. As it propagates through a material its electric and magnetic fields "disturb" the charged particles that it comes in contact with. These disturbances then cause the particles to radiate light at the same frequency, but with a phase shift. The sum of all these waves produced by the "disturbances" will lead to an electromagnetic wave with the same frequency as the original light, but with a shorter wavelength and, hence a slower speed.
Interesting, as fast as light moves, its path can be bent as it passes by regions in space with intense gravitational fields. This is fairly easily seen in galaxy clusters, which contain a lot of matter (including dark matter), which warps the path of light from more distant objects, such as quasars.
Lightspeed and Gravitational Waves
Current theories of physics predict that gravitational waves also travel at the speed of light, but this is still being confirmed as scientists study the phenomenon of gravitational waves from colliding black holes and neutron stars. Otherwise, there are no other objects that travel that fast. Theoretically, they can get close to the speed of light, but not faster.
One exception to this may be space-time itself. It appears that distant galaxies are moving away from us faster than the speed of light. This is a "problem" that scientists are still trying to understand. However, one interesting consequence of this is that a travel system based on the idea of a warp drive . In such a technology, a spacecraft is at rest relative to space and it's actually space that moves, like a surfer riding a wave on the ocean. Theoretically, this might allow for superluminal travel. Of course, there are other practical and technological limitations that stand in the way, but it's an interesting science-fiction idea that is getting some scientific interest.
Travel Times for Light
One of the questions that astronomers get from members of the public is: "how long would it take light to go from object X to Object Y?" Light gives them a very accurate way to measure the size of the universe by defining distances. Here are a few of the common ones distance measurements:
- The Earth to the Moon : 1.255 seconds
- The Sun to Earth : 8.3 minutes
- Our Sun to the next closest star : 4.24 years
- Across our Milky Way galaxy : 100,000 years
- To the closest spiral galaxy (Andromeda) : 2.5 million years
- Limit of the observable universe to Earth : 13.8 billion years
Interestingly, there are objects that are beyond our ability to see simply because the universe IS expanding, and some are "over the horizon" beyond which we cannot see. They will never come into our view, no matter how fast their light travels. This is one of the fascinating effects of living in an expanding universe.
Edited by Carolyn Collins Petersen
- Learn about the Doppler Effect
- Alpha Centauri: Gateway to the Stars
- Radiation in Space Gives Clues about the Universe
- Time Travel: Dream or Possible Reality?
- What is Matter?
- What is Blueshift?
- The Composition of the Universe
- Cold Dark Matter
- How Redshift Shows the Universe is Expanding
- How Meteors Form and What They Are
- What are Rotation and Revolution?
- Explore Johannes Kepler's Laws of Motion
- What Is Luminosity?
- Deciphering Star Charts for Skygazing
- Astronomy 101 - Learning About Stars
- Can Humans Hear Sound in Space?
Speed of Light [perfect visual explanations]
- Post author By M. Özgür Nevres
- Post date January 18, 2019
- No Comments on Speed of Light [perfect visual explanations]
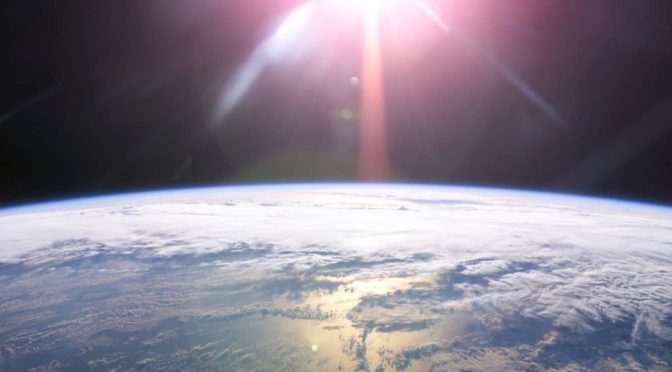
The speed of light is the Universal speed limit – nothing can travel faster than light . In the vacuum (commonly denoted c), its exact value is 299,792,458 meters per second (around 186,000 miles per second). In other words, if you could travel at the speed of light, you could go around the Earth 7.5 times in one second.
It might seem blazing fast, but, in fact, when you think of the vast distances between the celestial objects in the Universe, the speed of light is actually torturously slow.
For example, Alpha Centauri, the nearest star system to the Sun is 4.3 light-years away from Earth – the light emitted from them takes 4.3 years to reach us.
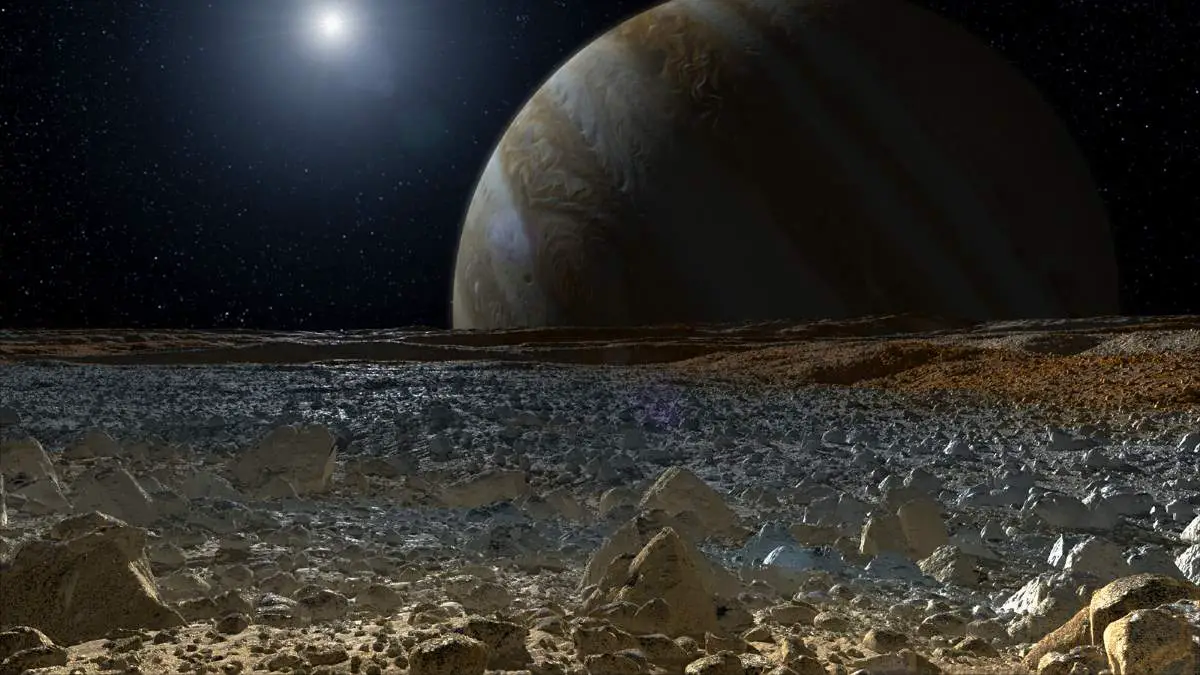
Related: Leaving Solar System at the Speed Of Light
Our Milky Way galaxy is around 150-200 thousand light-years in diameter. That means sending messages back and forth on either side of the galaxy would take hundreds of thousands of years. This is one of the reasons that there may be no Kardashev Type III civilization in the Universe (a civilization that can control its own galaxy – you can think of it as Isaac Asimov’s galactic empire in the Foundation series).
As Douglas Adams pointed out, “ Space is big . Really big. You just won’t believe how vastly, hugely, mind-bogglingly big it is.”
Even in our own solar system , the speed of light is so slow, communicating with spacecraft takes sometimes hours because of that. For example, it takes more than 21 hours for the signal to reach Voyager 1 (so it is more than 21 light hours away from the Earth).
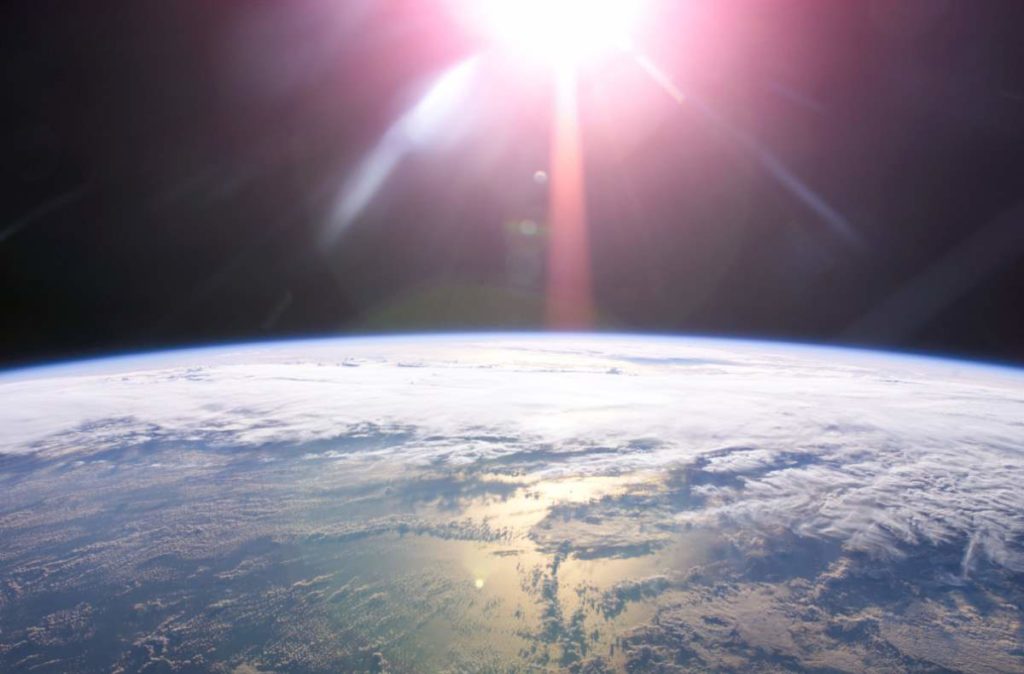
Speed of Light: see how torturously slow it is
To put things into perspective, NASA Goddard Planetary Scientist James O’ Donoghue created three animations to show how fast (or how slow) the speed of light is.
The first animation shows the light orbiting the Earth. The equatorial circumference of Earth is 40,075 km (24,901 miles). If our planet had no atmosphere (air refracts and slows downlight a little bit), a photon skimming along its surface could lap the equator nearly 7.5 times every second.
The second animation shows the light is traveling between the Earth and the moon. The average distance between the Earth and the moon is 384,400 km (238,855 miles). It takes a little more than a second for a photon to cover that distance.
The third animation shows the light traveling between the Earth and Mars. Now the speed of lights starts looking really slow. And this is just Mars, one of the closest planetary bodies to Earth.
Please note that In theory, the closest that Earth and Mars would approach each other would be when Mars is at its closest point to the sun (perihelion) and Earth is at its farthest (aphelion). This would put the planets only 33.9 million miles (54.6 million kilometers) apart. However, this has never happened in recorded history. The closest recorded approach of the two planets occurred in 2003 when they were only 34.8 million miles (56 million km) apart.
It would take around 140 hours to reach the edge of the solar system a photon emitted by the Sun – see the previous article titled “ Leaving the solar system at the speed of light “.
What Star Trek’s warp speeds would actually look like with real distance, in real-time
Dr. James O’Donoghue published another video showing what Star Trek’s warp speeds actually look like with real distance, in real-time.
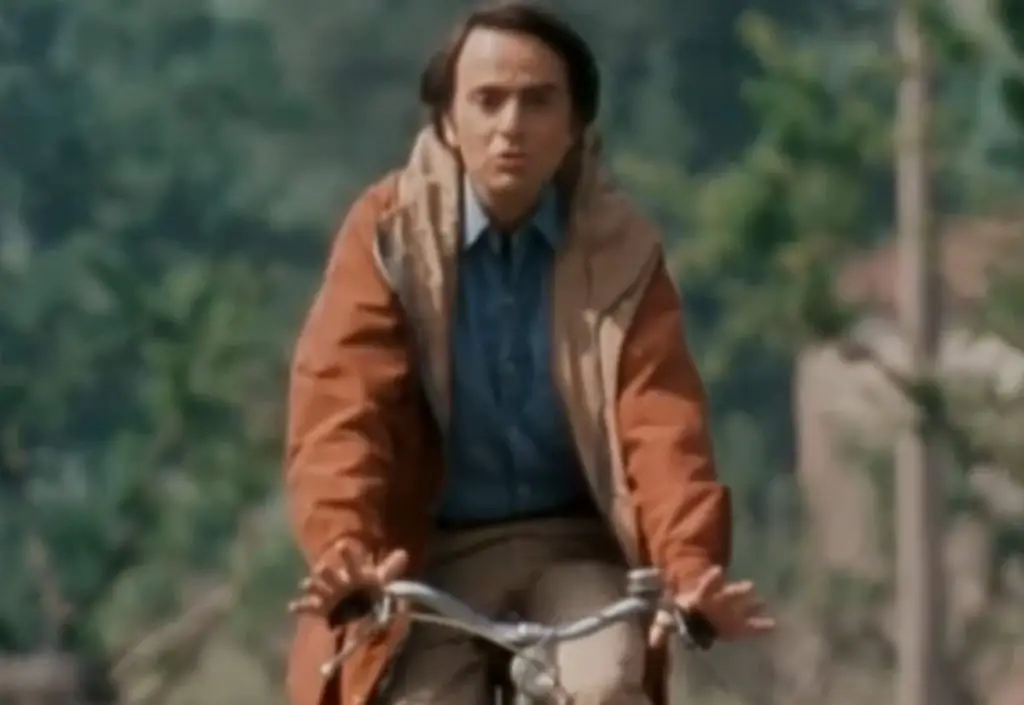
Related: Carl Sagan explains the speed of light while riding a bicycle
- How Fast Does Light Travel? | The Speed of Light on Space.com
- “The speed of light is torturously slow, and these 3 simple animations by a scientist at NASA prove it” on Business Insider
- The speed of Light on Wikipedia
- How Long Does It Take to Get to Mars? on Space.com
- Warp Drive on Wikipedia
- Recent Posts
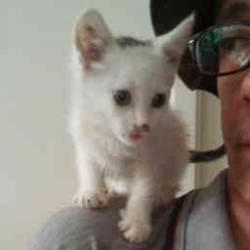
- Budget of NASA, Year by Year [1980-1989] - June 10, 2024
- Budget of NASA, Year by Year [1970-1979] - June 10, 2024
- Budget of NASA, Year by Year [1958-2024] - June 10, 2024
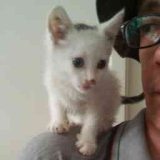
By M. Özgür Nevres
I am a software developer and a science enthusiast. I was graduated from the Istanbul Technical University (ITU), Computer Engineering. In the past, I worked at the Istanbul Technical University Science Center as a science instructor. I write about the planet Earth and science on this website, ourplnt.com. I am also an animal lover! I take care of stray cats & dogs. This website's all income goes directly to our furry friends. Please consider supporting me on Patreon , so I can help more animals!
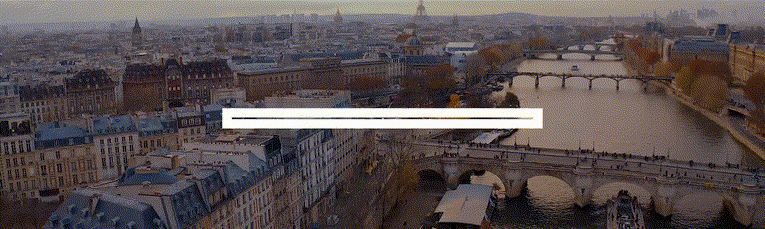
Leave a Reply Cancel reply
Your email address will not be published. Required fields are marked *
This site uses Akismet to reduce spam. Learn how your comment data is processed .

Get in Touch Get in Touch
- Shopping Cart
Speed of Light
What is the speed of light.
Somewhere in outer space, billions of light years from Earth, the original light associated with the Big Bang of the universe is blazing new ground as it continues moving outward. In stark contrast, another form of electromagnetic radiation originating on the Earth, radio waves from the inaugural live episode of The Lucy Show are broadcasting a premier somewhere in deep space, although greatly reduced in amplitude.
The basic concept behind both events involves the speed of light (and all other forms of electromagnetic radiation).

How Fast Is the Speed of Light
The speed of light, which scientists have thoroughly examined, is now expressed as a constant value denoted in equations by the symbol c. Not truly a constant, but rather the maximum speed in a vacuum, the speed of light in km, which is almost 300,000 kilometers per second, can be manipulated by changing media or with quantum interference.
Light traveling in a uniform substance, or medium, propagates in a straight line at a relatively constant speed, unless it is refracted, reflected, diffracted, or perturbed in some other manner. This well-established scientific fact is not a product of the Atomic Age or even the Renaissance, but was originally promoted by the ancient Greek scholar, Euclid, somewhere around 350 BC in his landmark treatise Optica. However, the intensity of light (and other electromagnetic radiation) is inversely proportional to the square of the distance traveled. Thus, after light has traveled twice a given distance, the intensity drops by a factor of four.
How Fast Is the Speed of Light in Air and Water?
When light traveling through the air enters a different medium, such as glass or water, the speed and wavelength of light are reduced (see Figure 2), although the frequency remains unaltered. Light travels at approximately 300,000 kilometers per second in a vacuum, which has a refractive index of 1.0, but it slows down to 225,000 kilometers per second in water (refractive index of 1.3; see Figure 2) and 200,000 kilometers per second in glass (refractive index of 1.5). In diamond, with a rather high refractive index of 2.4, the speed of light is reduced to a relative crawl (125,000 kilometers per second), being about 60 percent less than its maximum speed in a vacuum.
Because of the enormous journeys that light travels in outer space between galaxies (see Figure 1) and within the Milky Way, the expanse between stars is measured not in kilometers, but rather light-years, the distance light would travel in a year. A light-year equals 9.5 trillion kilometers or about 5.9 trillion miles. The distance from Earth to the next nearest star beyond our sun, Proxima Centauri, is approximately 4.24 light-years. By comparison, the Milky Way galaxy is estimated to be about 150,000 light-years in diameter, and the distance to the Andromeda galaxy is approximately 2.21 million light-years. This means that light leaving the Andromeda galaxy 2.21 million years ago is just arriving at Earth, unless it was waylaid by reflecting celestial bodies or refracting debris.
![light travel speed Image showing how fast the speed of light is when traveling through water, glass and a diamond]](https://static3.olympus-lifescience.com/data/olympusmicro/primer/lightandcolor/images/lightspeedfigure2.jpg?rev=B326)
When astronomers gaze into the night skies, they are observing a mixture of real time, the recent past, and ancient history. For example, during the period that pioneering Babylonians, Arab astrologers, and Greek astronomers described the stellar constellations, Scorpius (Scorpio to astrologers) still had the whiptail of a scorpion. The tail star and others in this constellation had appeared as novae in the skies between 500 and 1000 BC, but are no longer visible to today's stargazers. Although some of the stars that are observed in the night skies of Earth have long since perished, the light waves that carry their images are still reaching human eyes and telescopes. In effect, the light from their destruction (and the darkness of their absence) has not yet crossed the enormous distances of deep space because of insufficient time.
Early History of the Speed of Light
Empedocles of Acragas, who lived around 450 BC, was one of the first recorded philosophers to speculate that light traveled with a finite velocity. Almost a millennium later, around 525 AD, Roman scholar and mathematician Anicius Boethius attempted to document the speed of light, but after being accused of treason and sorcery, was decapitated for his scientific endeavors. Since the earliest application of black powder for fireworks and signals by the Chinese, man has wondered about the speed of light. With the flash of light and color preceding the explosive sound by several seconds, it did not require a serious calculation to realize that the speed of light obviously exceeded the speed of sound.
Speed of Light in Transparent Materials
Discover how the speed of light is reduced in proportion to the refractive index of a material when the light enters a new transparent medium, such as air, water, or glass.
The Chinese secrets behind explosives made their way to the West during the middle of the Thirteenth Century, and with them, came questions about the speed of light. Prior to this period, other investigators must have considered the flash of lightning followed later by the clap of thunder, typical of a thunderstorm, but offered no plausible scientific explanations about the nature of the delay. The Arabic scholar Alhazen was the first serious optical scientist to suggest (around 1000 AD) that light had a finite speed, and by 1250 AD, British optics pioneer Roger Bacon wrote that the speed of light was finite, although very rapid. Still, the widely held opinion by a majority of scientists during this period was that the speed of light is infinite and could not be measured.
In 1572, the famous Danish astronomer Tycho Brahe was the first to describe a supernova, which occurred in the constellation Cassiopeia. After watching a "new star" suddenly appear in the sky, slowly intensify in brightness, and then fade from view over an 18-month period, the astronomer was mystified, but intrigued. These novel celestial visions drove Brahe and his contemporaries to question the widely held notion of a perfect and unchanging universe having an infinite speed of light. The belief that light has infinite speed was hard to displace, although a few scientists were beginning to question the speed of light in the Sixteenth Century. As late as 1604, the German physicist Johannes Kepler speculated that the speed of light was instantaneous. He added in his published notes that the vacuum of space did not slow the speed of light down, hampering, to a limited degree, the quest by his contemporaries for the ether that supposedly filled space and carried the light.

Ole Roemer’s Speed of Light Estimations
Shortly after the invention and some relatively crude refinements to the telescope, Danish astronomer Ole Roemer (in 1676) was the first scientist to make a rigorous attempt to estimate the speed of light. By studying Jupiter's moon Io and its frequent eclipses, Roemer was able to predict the periodicity of an eclipse period for the moon (Figure 3). However, after several months, he noticed that his predictions were slowly becoming less accurate by progressively longer time intervals, reaching a maximum error of about 22 minutes (a rather large discrepancy, considering how far light travels in that time span). Then, just as oddly, his predictions again became more accurate over several months, with the cycle repeating itself. Working at the Paris Observatory, Roemer soon realized that the observed differences were caused by variations in the distance between the Earth and Jupiter, due to orbital pathways of the planets. As Jupiter moved away from the Earth, light had a longer distance to travel, taking additional time to reach the Earth. Applying the relatively inaccurate calculations for the distances between Earth and Jupiter available during the period, Roemer was able to estimate the speed of light at about 137,000 miles (or 220,000 kilometers) per second. Figure 3 illustrates a reproduction of the original drawings by Roemer delineating his methodology utilized to determine the speed of light.
Roemer's work stirred the scientific community, and many investigators began to reconsider their speculations about the infinite speed of light. Sir Isaac Newton, for example, wrote in his landmark 1687 treatise Philosophiae Naturalis Prinicipia Mathematica (Mathematical Principles of Natural Philosophy), "For it is now certain from the phenomena of Jupiter's satellites, confirmed by the observations of different astronomers, that light is propagated in succession and requires about seven or eight minutes to travel from the sun to the earth", which is actually a remarkably close estimate for the correct speed of light. Newton's respected opinion and widespread reputation was instrumental in jump-starting the Scientific Revolution, and helped launch new research by scientists who now endorsed light's speed as finite.
James Bradley’s Speed of Light Estimations
The next in line to provide a useful estimate of the speed of light was the British physicist James Bradley. In 1728, a year after Newton's death, Bradley estimated the speed of light in a vacuum to be approximately 301,000 kilometers per second, using stellar aberrations. These phenomena are manifested by an apparent variation in the position of stars due to the motion of the Earth around the sun. The degree of stellar aberration can be determined from the ratio of the Earth's orbital speed to the speed of light. By measuring the stellar aberration angle and applying that data to the orbital speed of the Earth, Bradley was able to arrive at a remarkably accurate estimate.
In 1834, Sir Charles Wheatstone, inventor of the kaleidoscope and a pioneer in the science of sound, attempted to measure the speed of electricity. Wheatstone invented a device that utilized rotating mirrors and capacitative discharge through a Leyden jar to generate and clock the movement of sparks through almost eight miles of wire. Unfortunately, his calculations (and perhaps his instrumentation) were in error to such a degree that Wheatstone estimated the velocity of electricity at 288,000 miles per second, a mistake that led him to believe that electricity traveled faster than light. Wheatstone's research was later expanded upon by French scientist Dominique François Jean Arago. Although he failed to complete his work before his eyesight failed in 1850, Arago correctly postulated that light traveled slower in water than air.
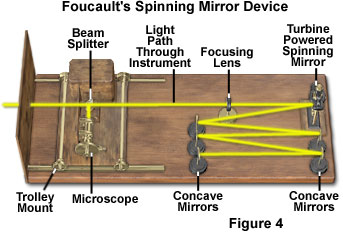
Fizeau and Foucault’s Speed of Light Experiments
Meanwhile in France, rival scientists Armand Fizeau and Jean-Bernard-Leon Foucault independently attempted to measure the speed of light, without relying on celestial events, by taking advantage of Arago's discoveries and expanding on Wheatstone's rotating mirror instrument design. In 1849, Fizeau engineered a device that flashed a light beam through a toothed wheel (instead of a rotating mirror), and then onto a fixed mirror positioned at a distance of 5.5 miles away. By rotating the wheel at a rapid rate, he was able to steer the beam through a gap between two of the teeth on the outward journey and catch reflected rays in the neighboring gap on the way back. Armed with the wheel speed and distance traveled by the pulsed light, Fizeau was able to calculate the speed of light. He also discovered that light travels faster in air than in water (confirming Arago's hypothesis), a fact that fellow countryman Foucault later confirmed through experimentation.
Foucault employed a rapidly rotating mirror driven by a compressed air turbine to measure the speed of light. In his apparatus (see Figure 4), a narrow beam of light is passed through an aperture and then through a glass window (acting also as a beamsplitter) with a finely graduated scale before impacting on the rapidly spinning mirror. Light reflected from the spinning mirror is directed through a battery of stationary mirrors in a zigzag pattern designed to increase the path length of the instrument to about 20 meters without a corresponding increase in size. In the amount of time it took the light to reflect through the series of mirrors and return to the rotating mirror, a slight shift in the mirror position had occurred. Subsequently, light reflected from the shifted position of the spinning mirror follows a new pathway back to the source and into a microscope mounted on the instrument. The tiny shift in light could be seen through the microscope and recorded. By analysis of the data collected from his experiment, Foucault was able to calculate the speed of light as 298,000 kilometers per second (approximately 185,000 miles per second).
The light path in Foucault's device was short enough to be utilized in the measurement of light speeds through media other than air. He discovered that the speed of light in water or glass was only about two-thirds of the value in air, and he also concluded that the speed of light through a given medium is inversely proportional to the refractive index. This remarkable result is consistent with the predictions about light behavior developed hundreds of years earlier from the wave theory of light propagation.
Michelson and Morley’s Speed of Light Apparatus
Following Foucault's lead, a Polish-born American physicist named Albert A. Michelson attempted to increase the accuracy of the method, and successfully measured the speed of light in 1878 with a more sophisticated version of the apparatus along a 2,000-foot wall lining the banks of England's Severn River. Investing in high quality lenses and mirrors to focus and reflect a beam of light over a much longer pathway than the one utilized by Foucault, Michelson calculated a final result of 186,355 miles per second (299,909 kilometers per second), allowing for a possible error of about 30 miles per second. Due to the increased sophistication of his experimental design, the accuracy of Michelson's measurement was over 20 times greater than Foucault's.
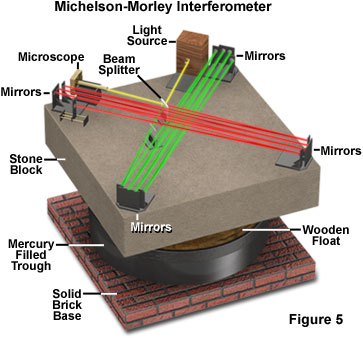
During the late 1800s it was still believed by most scientists that light propagates through space utilizing a carrier medium termed the ether. Michelson teamed with scientist Edward Morley in 1887 to devise an experimental method for detecting the ether by observing relative changes in the speed of light as the Earth completed its orbit around the sun. In order to accomplish this goal, they designed an interferometer that splits a beam of light and re-directs the individual beams through two different pathways, each over 10 meters in length, using a complex array of mirrors. Michelson and Morley reasoned that if the Earth is traveling through an ether medium, the beam reflecting back and forth perpendicular to the flow of ether would have to travel farther than the beam reflecting parallel to the ether. The result would be a delay in one of the light beams that could be detected when the beams were recombined through interference.
The experimental apparatus built by Michelson and Morley was massive (see Figure 5). Mounted on a slowly rotating stone slab that was over five feet square and 14 inches thick, the instrument was further protected by an underlying pool of mercury that acted as a frictionless shock absorber to remove vibrations from the Earth. Once the slab was set into motion, achieving a top speed of 10 revolutions per hour, it took hours to reach a halt again. Light passing through a beamsplitter, and reflected by the mirror system, was examined with a microscope for interference fringes, but none were ever observed. However, Michelson utilized his interferometer to accurately determine the speed of light at 186,320 miles per second (299,853 kilometers per second), a value that stood as the standard for the next 25 years. The failure to detect a change in the speed of light by the Michelson-Morley experiment set in motion the beginnings of an end to the ether controversy, which was finally laid to rest by the theories of Albert Einstein in the early Twentieth Century.
Einstein’s Special Theory of Relativity and the Speed of Light
In 1905, Einstein published his Special Theory of Relativity followed by the General Theory of Relativity in 1915. The first theory related to the movement of objects at constant velocity relative to one another, while the second focused on acceleration and its links with gravity. Because they challenged many long-standing hypotheses, such as Isaac Newton's law of motion, Einstein's theories were a revolutionary force in physics. The idea of relativity embodies the concept that the velocity of an object can be determined only relative to the position of the observer. As an example, a man walking inside an airliner appears to be traveling at about one mile per hour in the reference frame of the aircraft (which itself is moving at 600 miles per hour). However, to an observer on the ground, the man seems to be moving at 601 miles per hour.
Einstein assumed in his calculations that the speed of light traveling between two frames of reference remains the same for observers in both locations. Because an observer in one frame uses light to determine the position and velocity of objects in another frame, this changes the manner in which the observer can relate the position and velocity of the objects. Einstein employed this concept to derive several important formulas describing how objects in one frame of reference appear when viewed from another that is in uniform motion relative to the first. His results led to some unusual conclusions, although the effects only become noticeable when the relative velocity of an object approaches the speed of light. In summary, the major implications of Einstein's fundamental theories and his often-referenced relativity equation:
can be summarized as follows:
The length of an object decreases, relative to an observer, as the velocity of that object increases.
When a frame of reference is moving, time intervals become shorter. In other words, a space traveler moving at or near the speed of light could leave the Earth for many years, and return having experienced a time lapse of only a few months.
The mass of a moving object increases with its velocity, and as the velocity approaches the speed of light, the mass approaches infinity. For this reason, it is widely believed that travel faster than the speed of light is impossible, because an infinite amount of energy would be required to accelerate an infinite mass.
Although Einstein's theory affected the entire world of physics, it had particularly important implications for those scientists who were studying light. The theory explained why the Michelson-Morley experiment failed to produce the expected results, discouraging further serious scientific investigations into the nature of ether as a carrier medium. It also demonstrated that nothing can move faster than the speed of light in a vacuum, and that this speed is a constant and unchanging value. Meanwhile, experimental scientists continued to apply increasingly sophisticated instruments to zero in on a correct value for the speed of light and reduce the error in its measurement.
Measurements of the Speed of Light
During the late Nineteenth Century, advances in radio and microwave technology provided novel approaches for measuring the speed of light. In 1888, more than 200 years after Roemer's pioneering celestial observations, German physicist Heinrich Rudolf Hertz measured the speed of radio waves. Hertz arrived at a value near 300,000 kilometers per second, confirming James Clerk Maxwell's theory that radio waves and light were both forms of electromagnetic radiation. Additional proof was gathered during the 1940s and 1950s, when British physicists Keith Davy Froome and Louis Essen employed radio and microwaves, respectively, to more precisely measure the speed of electromagnetic radiation.
Maxwell is also credited with defining the speed of light and other forms of electromagnetic radiation, not through measurement, but by mathematical deduction. During his research attempts to find a link between electricity and magnetism, Maxwell theorized that a changing electrical field produces a magnetic field, the reverse corollary of Faraday's law. He proposed that electromagnetic waves are composed of combined oscillating electric and magnetic waves, and calculated the velocity of these waves through space as:
where ε is the permitivity and µ is the permeability of free space, two constants that can be measured with a relatively high degree of accuracy. The result is a value that closely approximates the measured speed of light.
In 1891, continuing his studies on the speed of light and astronomy, Michelson created a large-scale interferometer using the refracting telescope at the Lick Observatory in California. His observations were based on the delay in the arrival time of light when viewing distant objects, such as stars, which can be quantitatively analyzed to measure both the size of celestial bodies and the speed of light. Almost 30 years later, Michelson moved his experiments to the Mount Wilson Observatory, and applied the same techniques to the 100-inch telescope, the world's largest at the time.
By incorporating an octagonal rotating mirror into his experimental design, Michelson arrived at a value of 299,845 kilometers per second for the speed of light. Although Michelson died before completing his experiments, his co-worker at Mount Wilson, Francis G. Pease, continued to employ the innovative technique to conduct research into the 1930s. Using a modified interferometer, Pease made numerous measurements over several years and finally determined that the correct value for the speed of light is 299,774 kilometers per second, the closest measurement achieved to that date. Several years later, in 1941, the scientific community set a standard for the speed of light. This value, 299,773 kilometers per second, was based on a compilation from the most accurate measurements of the period. Figure 6 presents a graphical representation of light speed measurements over the past 200 years.
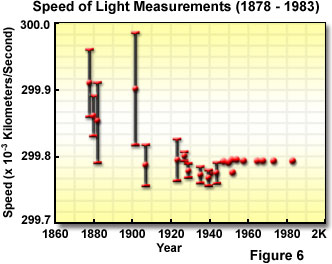
By the late 1960s, lasers were becoming stable research tools with highly defined frequencies and wavelengths. It quickly became obvious that a simultaneous measurement of frequency and wavelength would yield a very accurate value for the speed of light, similar to an experimental approach carried out by Keith Davy Froome using microwaves in 1958. Several research groups in the United States and in other countries measured the frequency of the 633-nanometer line from an iodine-stabilized helium-neon laser and obtained highly accurate results. In 1972, the National Institute of Standards and Technology employed the laser technology to measure the speed at 299,792,458 meters per second (186,282 miles per second), which ultimately resulted in the redefinition of the meter through a highly accurate estimate for the speed of light.
Starting with Roemer's 1676 breakthrough endeavors, the speed of light has been measured at least 163 times utilizing a wide variety of different techniques by more than 100 investigators (see Table 1 for a compilation of methods, investigators, and dates). As scientific methods and devices were refined, the error limits of the estimates narrowed, although the speed of light has not significantly changed since Roemer's seventeenth century calculations. In 1983, more than 300 years after the first serious measurement attempt, the speed of light was defined as being 299,792.458 kilometers per second by the Seventeenth General Congress on Weights and Measures. Thus, the meter is defined as the distance light travels during a time interval of 1/299,792,458 seconds. In general, however, (even in many scientific calculations) the speed of light is rounded to 300,000 kilometers (or 186,000 miles) per second. Arriving at a standard value for the speed of light was important for establishing an international system of units that would enable scientists from around the world to compare their data and calculations.
There is a mild controversy over whether evidence exists that the speed of light has been slowing since the time of the Big Bang, when it may have moved significantly faster, as suggested by some investigators. Although arguments presented and countered perpetuate this debate, most scientists still contend that the speed of light is a constant. Physicists point out that the actual speed of light as measured by Roemer and his followers has not significantly changed, but rather point to a series of refinements in scientific instrumentation associated with increases in precision of the measurements utilized to establish the speed of light. Today, the distance between Jupiter and the Earth is known with a high degree of accuracy, as are the diameter of the solar system and the orbital trajectories of the planets. When researchers apply this data to rework the calculations made over the past few centuries, they derive values for the speed of light comparable to those obtained with more modern and sophisticated instrumentation.
Contributing Authors
Kenneth R. Spring - Scientific Consultant, Lusby, Maryland, 20657.
Thomas J. Fellers , Lawrence D. Zuckerman , and Michael W. Davidson - National High Magnetic Field Laboratory, 1800 East Paul Dirac Dr., The Florida State University, Tallahassee, Florida, 32310.
Not Available in Your Country
Sorry, this page is not available in your country.
- Contact Us Contact Us
- Subscribe to Mailing List Subscribe to Mailing List
How "Fast" is the Speed of Light?
Light travels at a constant, finite speed of 186,000 mi/sec. A traveler, moving at the speed of light, would circum-navigate the equator approximately 7.5 times in one second. By comparison, a traveler in a jet aircraft, moving at a ground speed of 500 mph, would cross the continental U.S. once in 4 hours.
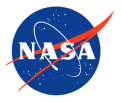
Suggested Searches
- Climate Change
- Expedition 64
- Mars perseverance
- SpaceX Crew-2
- International Space Station
- View All Topics A-Z
Humans in Space
Earth & climate, the solar system, the universe, aeronautics, learning resources, news & events.
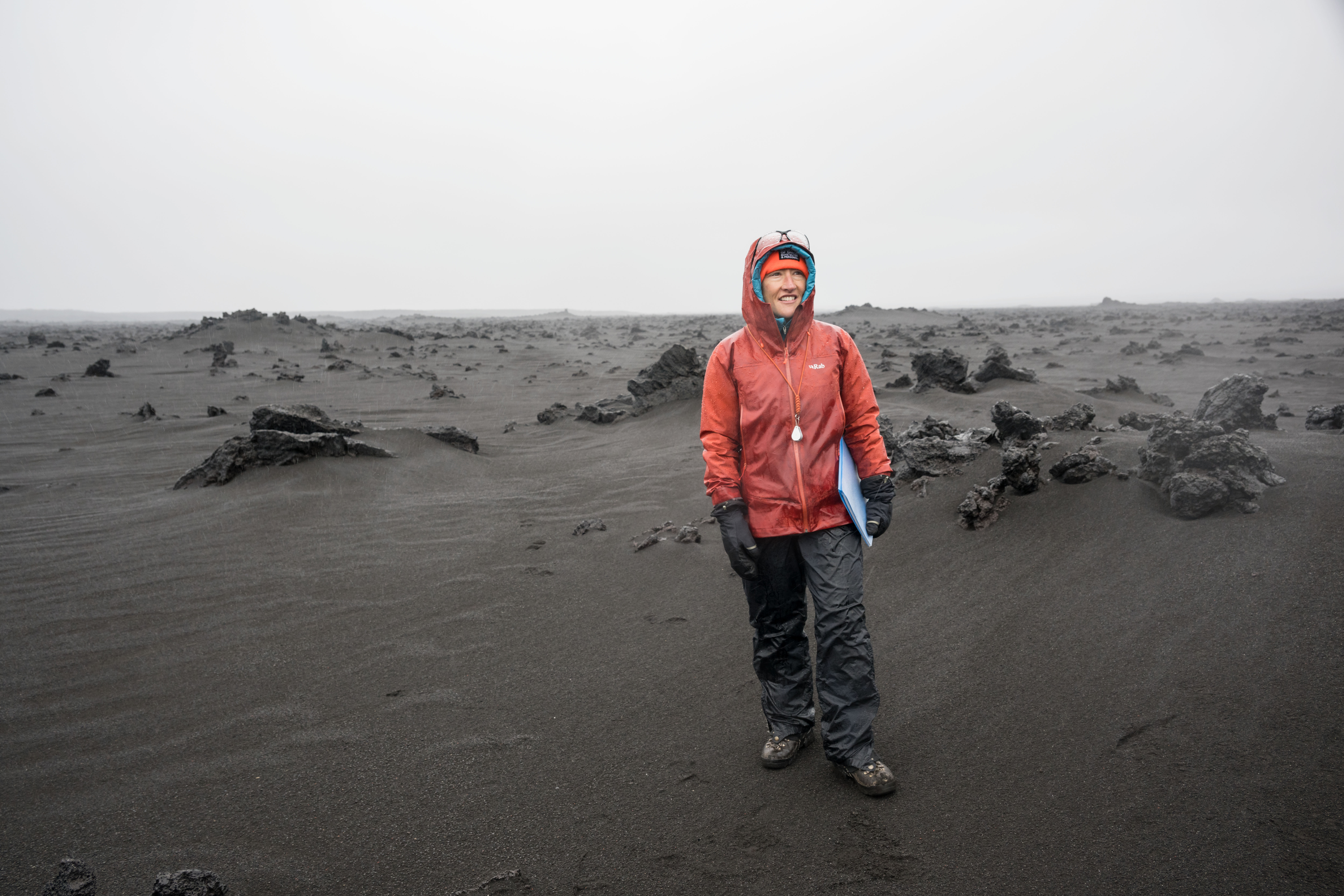
NASA’s Artemis II Crew Uses Iceland Terrain for Lunar Training
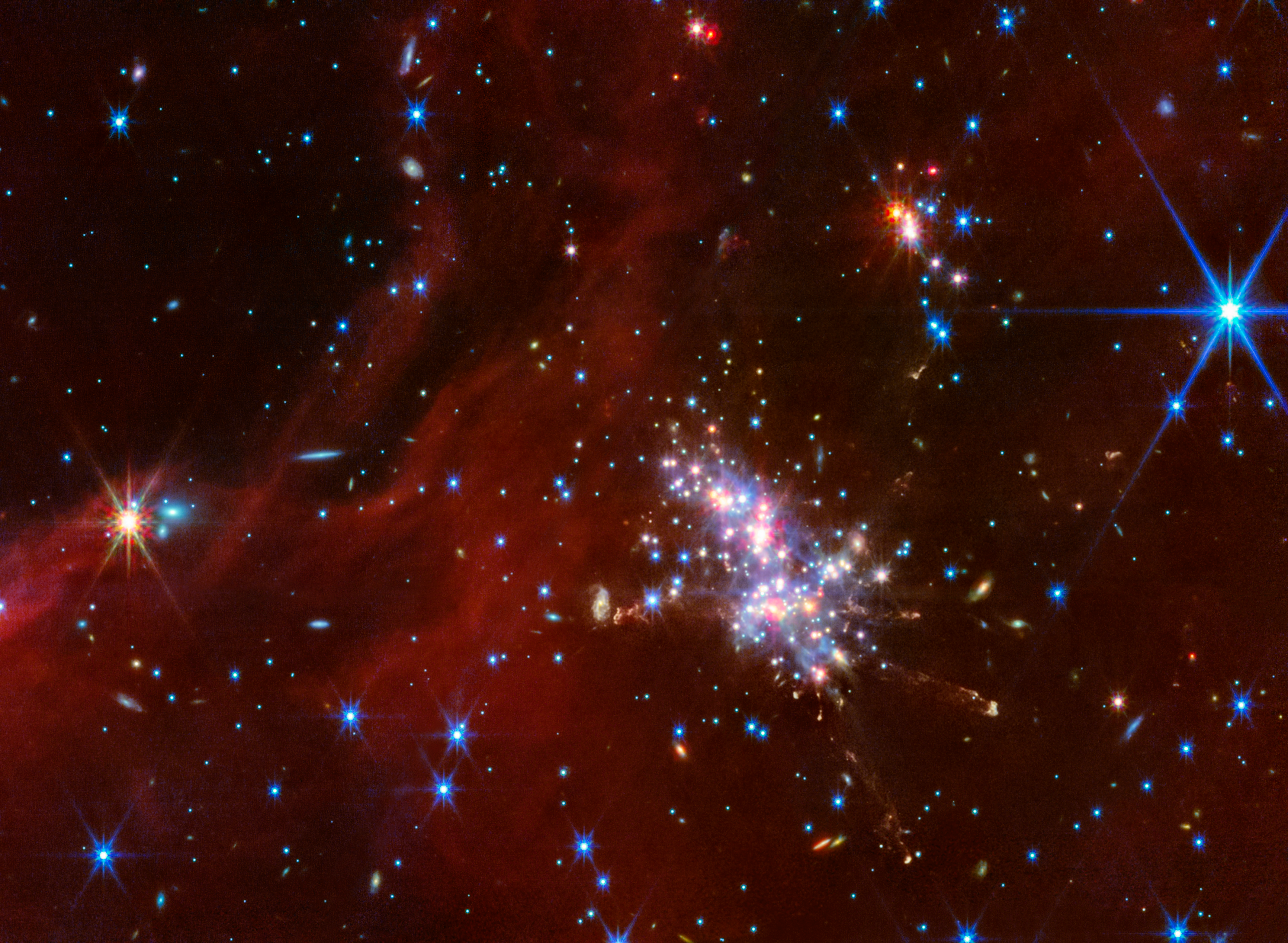
NASA’s Webb Peers into the Extreme Outer Galaxy

What’s Up: September 2024 Skywatching Tips from NASA
- Search All NASA Missions
- A to Z List of Missions
- Upcoming Launches and Landings
- Spaceships and Rockets
- Communicating with Missions
- James Webb Space Telescope
- Hubble Space Telescope
- Why Go to Space
- Commercial Space
- Destinations
- Living in Space
- Explore Earth Science
- Earth, Our Planet
- Earth Science in Action
- Earth Multimedia
- Earth Science Researchers
- Pluto & Dwarf Planets
- Asteroids, Comets & Meteors
- The Kuiper Belt
- The Oort Cloud
- Skywatching
- The Search for Life in the Universe
- Black Holes
- The Big Bang
- Dark Energy & Dark Matter
- Earth Science
- Planetary Science
- Astrophysics & Space Science
- The Sun & Heliophysics
- Biological & Physical Sciences
- Lunar Science
- Citizen Science
- Astromaterials
- Aeronautics Research
- Human Space Travel Research
- Science in the Air
- NASA Aircraft
- Flight Innovation
- Supersonic Flight
- Air Traffic Solutions
- Green Aviation Tech
- Drones & You
- Technology Transfer & Spinoffs
- Space Travel Technology
- Technology Living in Space
- Manufacturing and Materials
- Science Instruments
- For Kids and Students
- For Educators
- For Colleges and Universities
- For Professionals
- Science for Everyone
- Requests for Exhibits, Artifacts, or Speakers
- STEM Engagement at NASA
- NASA's Impacts
- Centers and Facilities
- Directorates
- Organizations
- People of NASA
- Internships
- Our History
- Doing Business with NASA
- Get Involved
NASA en Español
- Aeronáutica
- Ciencias Terrestres
- Sistema Solar
- All NASA News
- Video Series on NASA+
- Newsletters
- Social Media
- Media Resources
- Upcoming Launches & Landings
- Virtual Guest Program
- Image of the Day
- Sounds and Ringtones
- Interactives
- STEM Multimedia
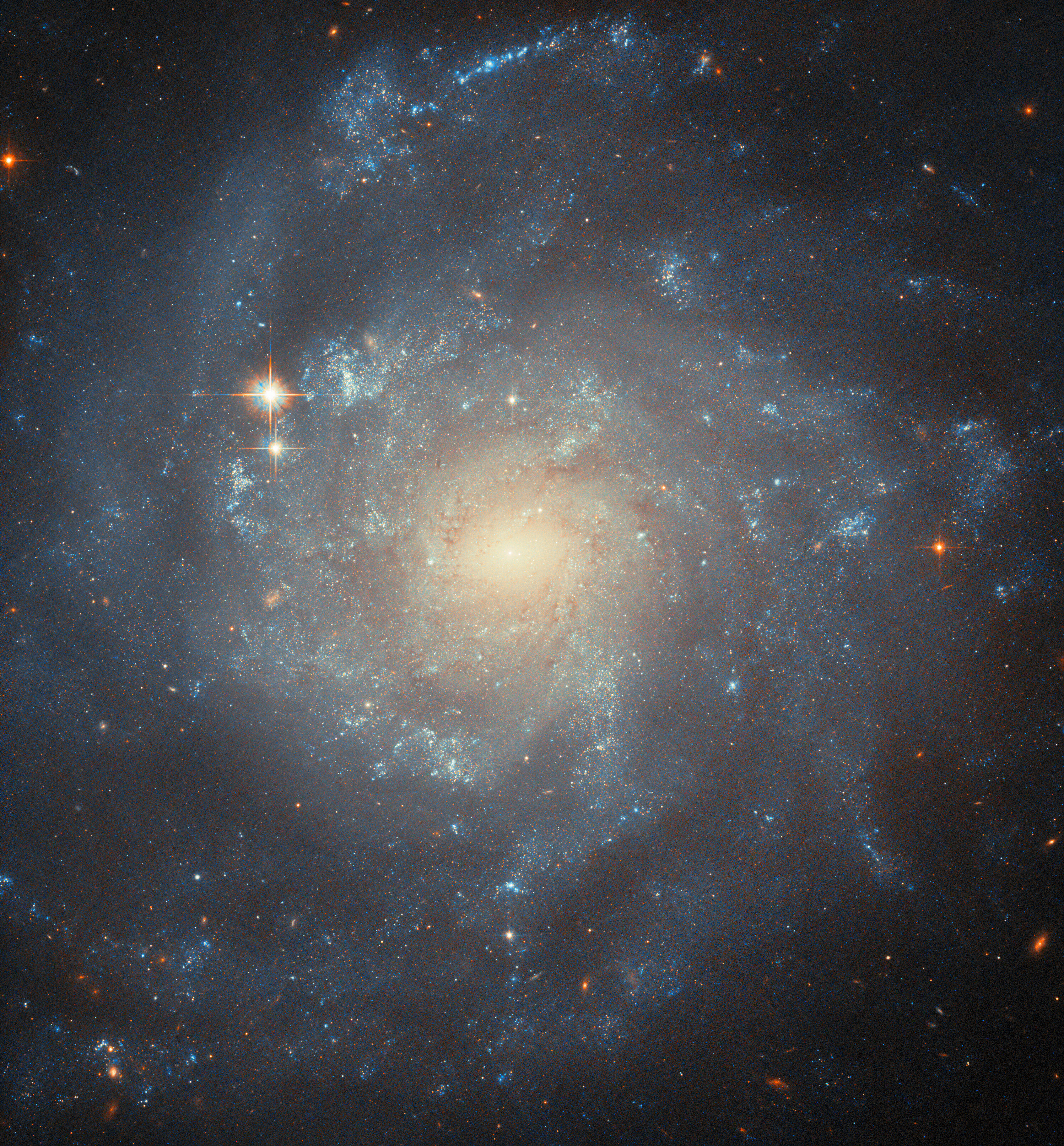
Hubble Examines a Spiral Star Factory
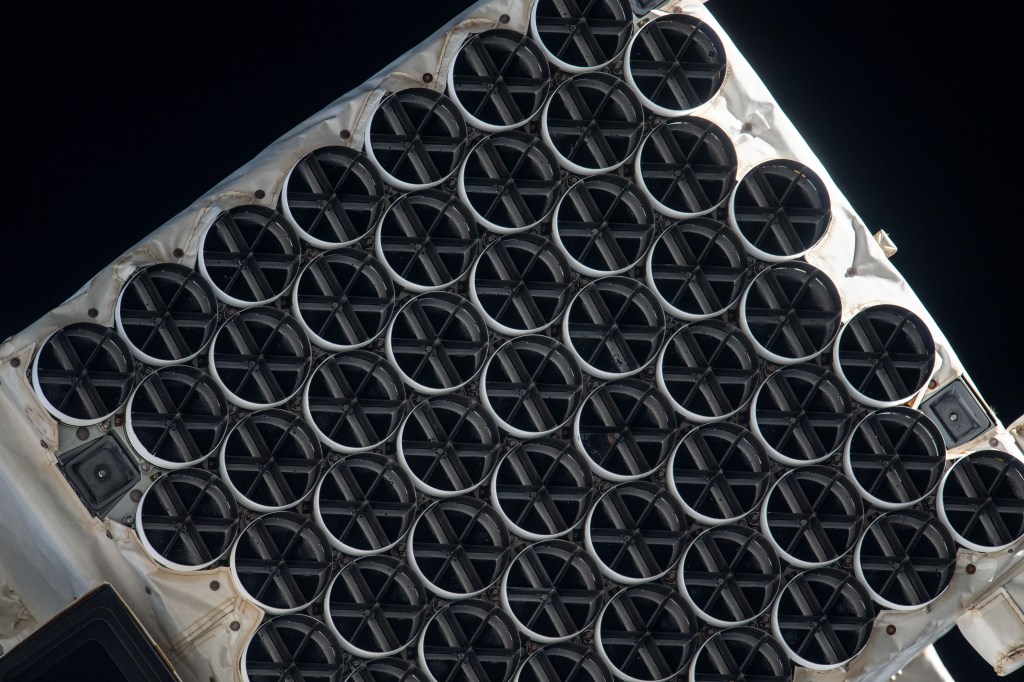
NASA’s SpaceX Crew-9 to Conduct Space Station Research
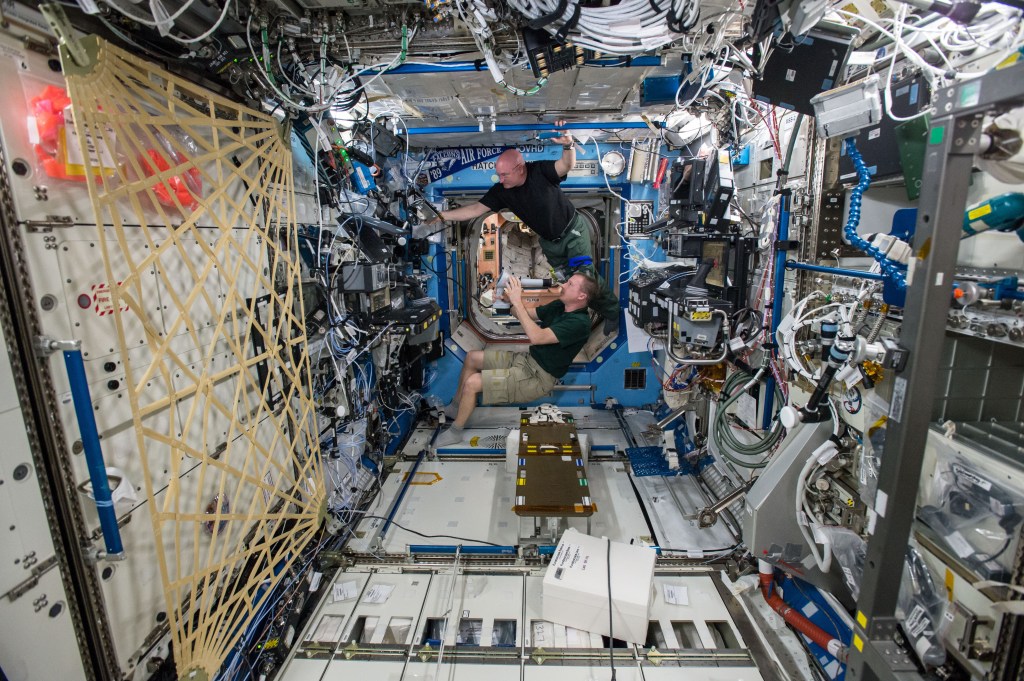
Station Science Top News: September 13, 2024
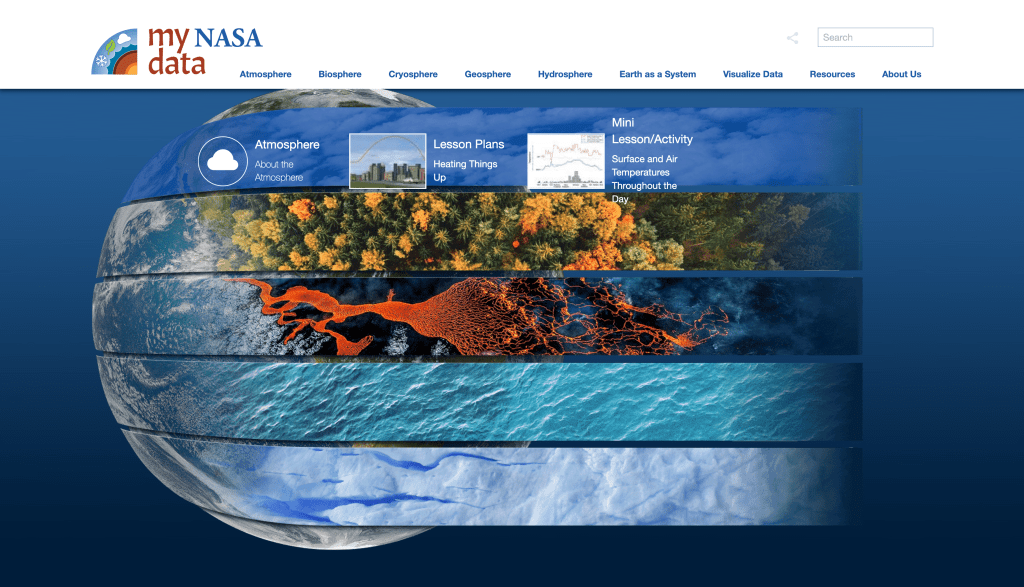
Going Back-to-School with NASA Data
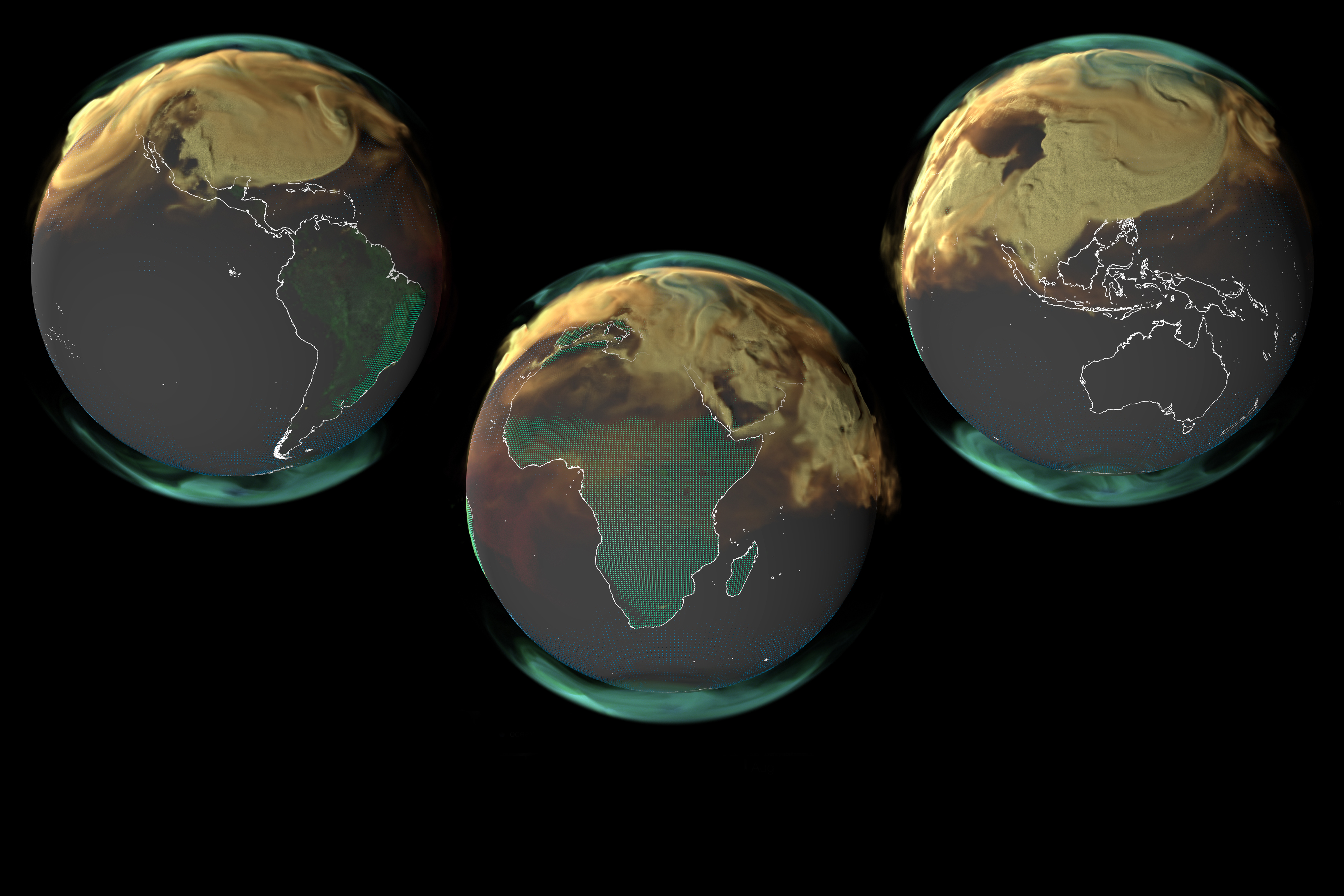
Amendment 48: A.5 Carbon Cycle Science Final Text and Due Dates.

NASA Finds Summer 2024 Hottest to Date
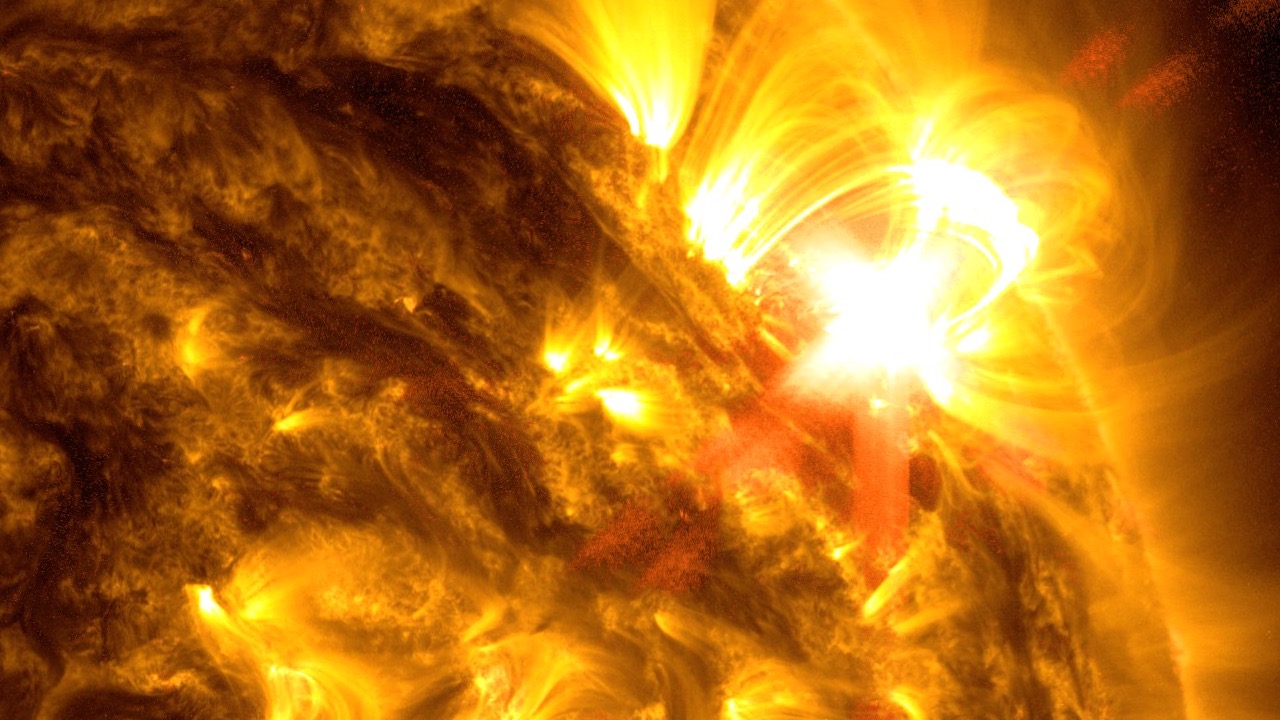
Solar Storms and Flares
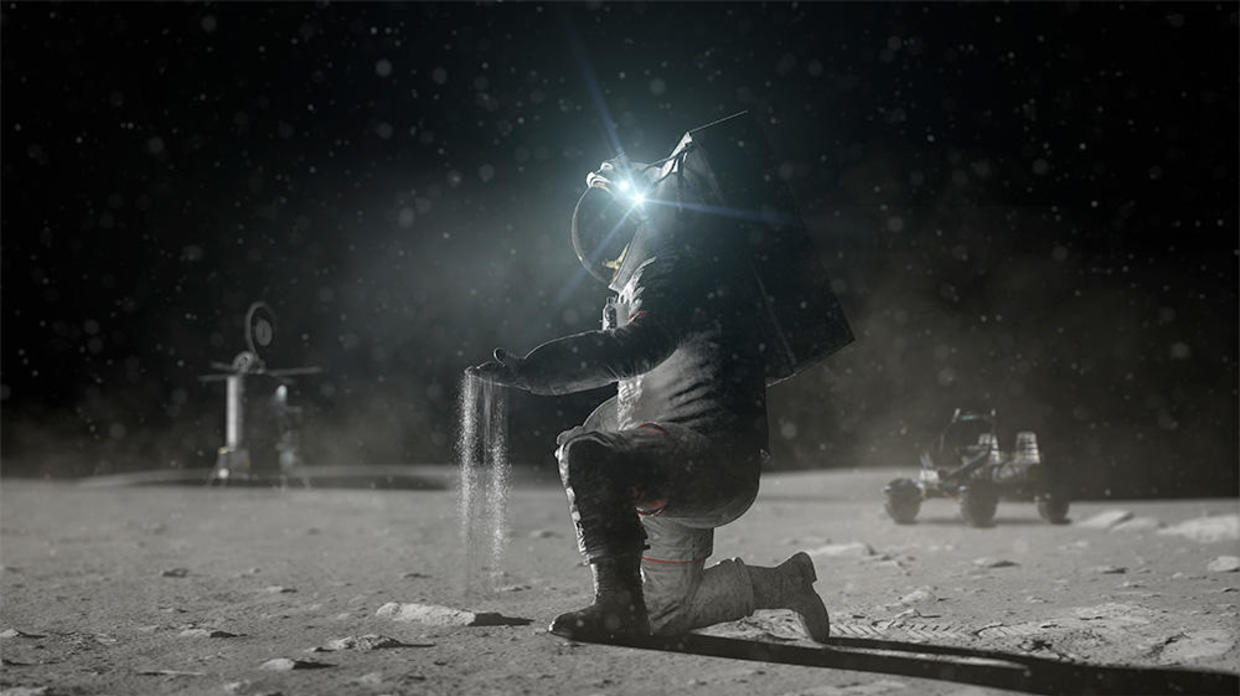
Amendment 47: DRAFT F.12 Artemis IV Deployed Instruments Program Released for Community Comment.
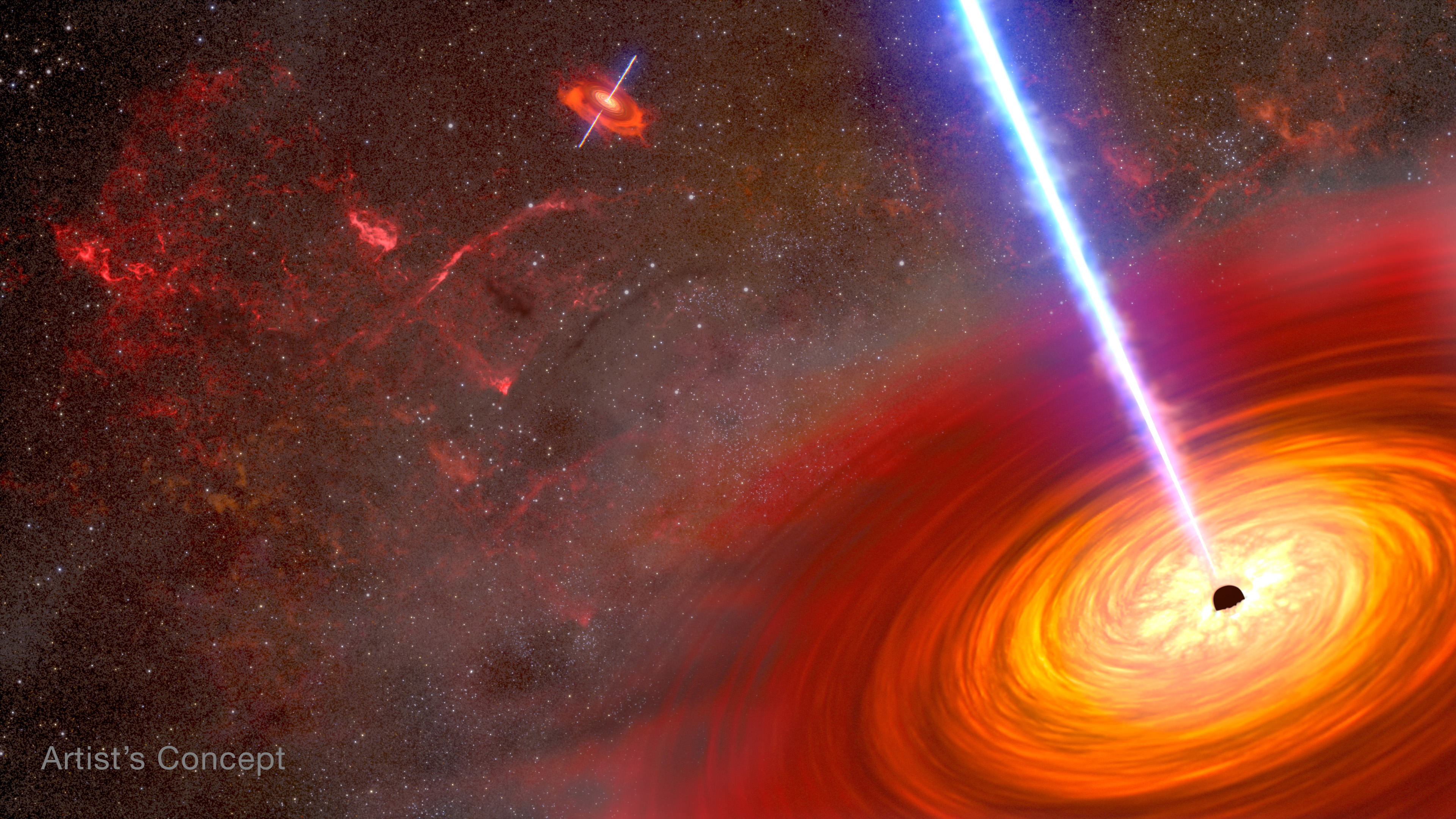
NASA’s Hubble, Chandra Find Supermassive Black Hole Duo
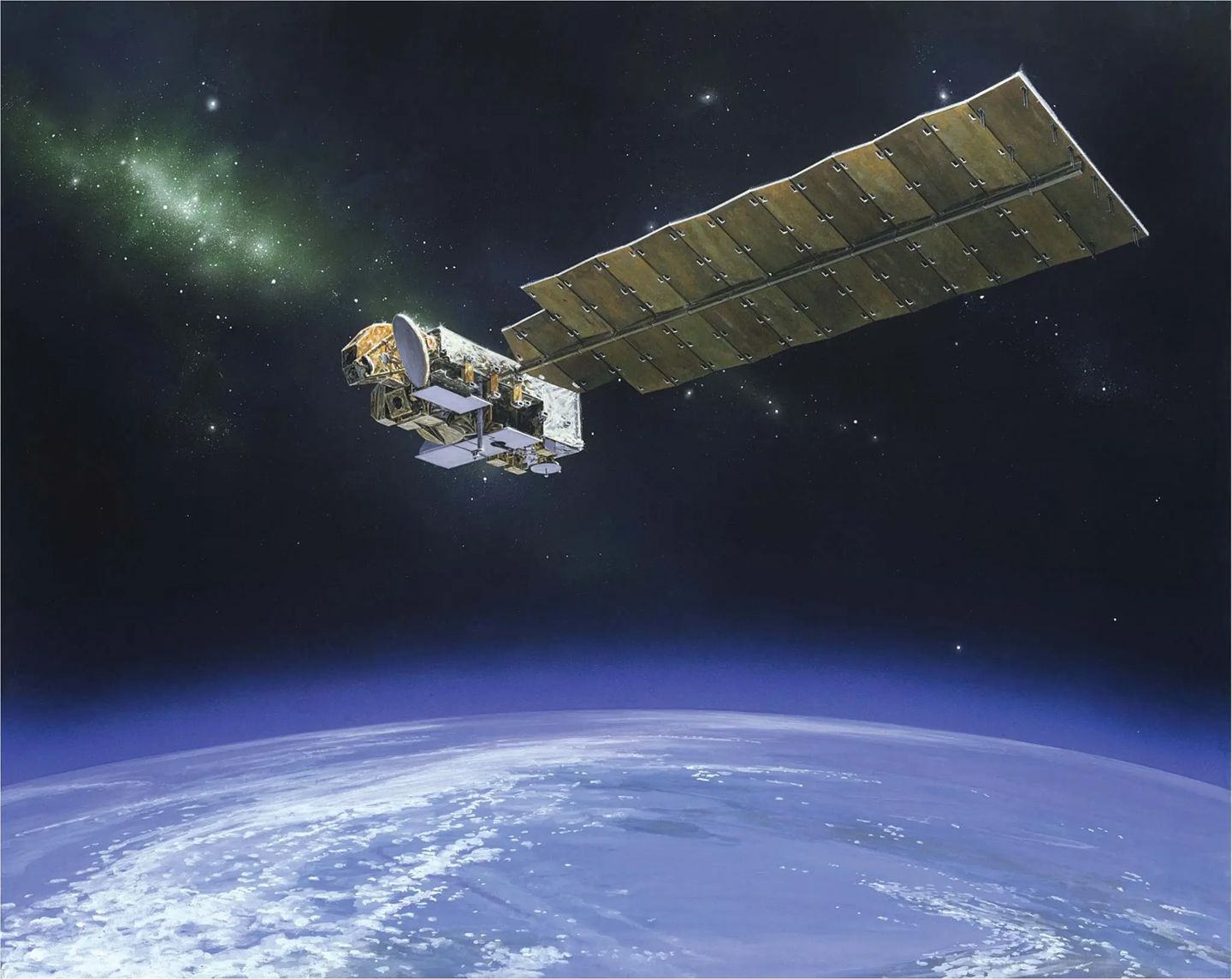
Aura at 20 Years
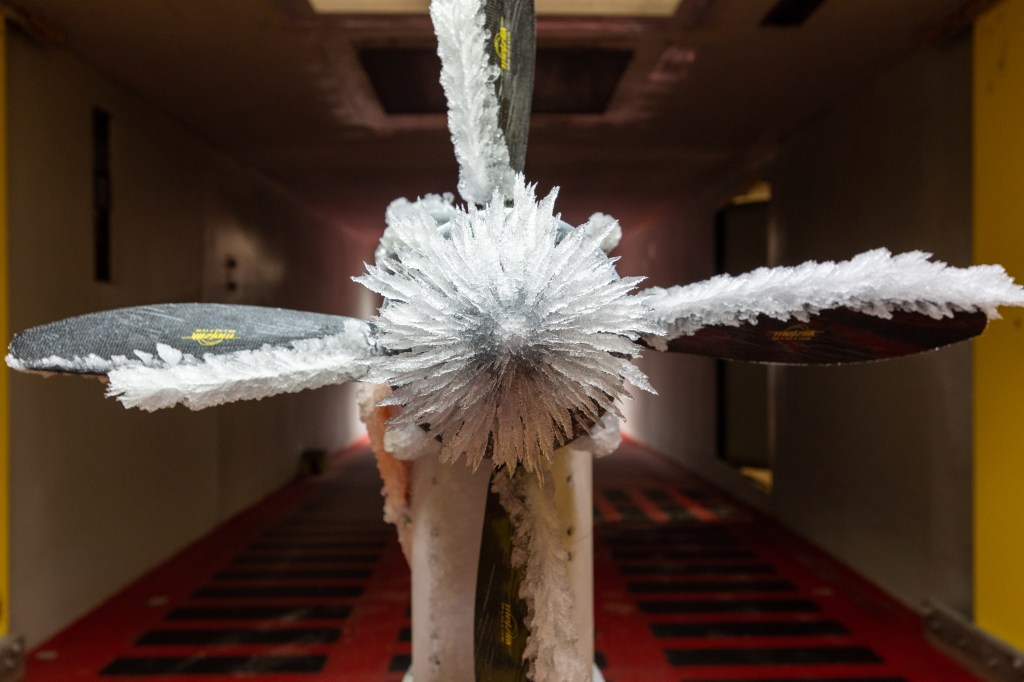
NASA Tunnel Generates Decades of Icy Aircraft Safety Data

Research Plane Dons New Colors for NASA Hybrid Electric Flight Tests
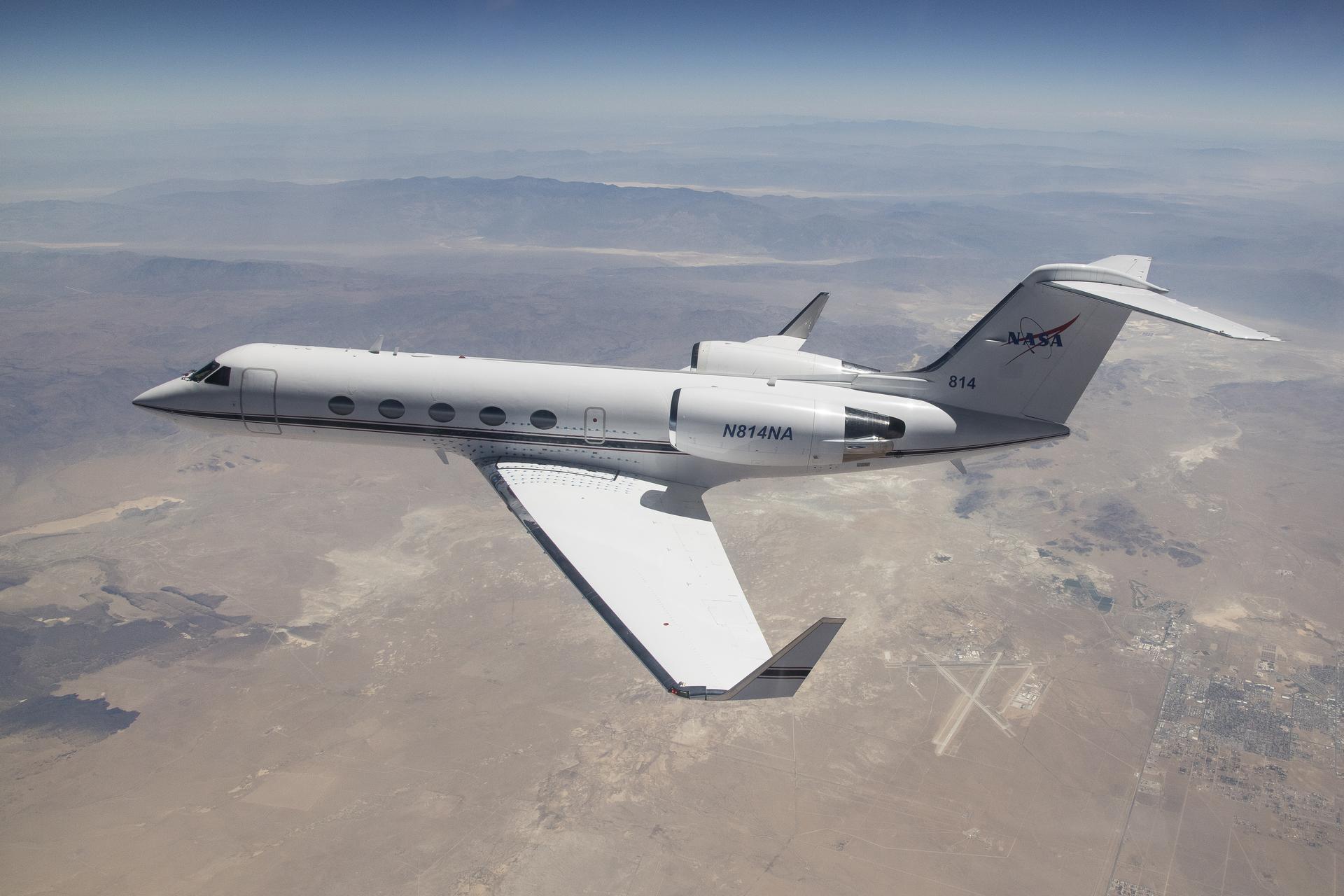
NASA G-IV Plane Will Carry Next-Generation Science Instrument

SCaN Lunar Support

Printed Engines Propel the Next Industrial Revolution

NASA Mobilizes Resource for HBCU Scholars, Highlighted at Conference

NASA Moon to Mars Architecture Art Challenge

15 Years Ago: Japan launches HTV-1, its First Resupply Mission to the Space Station

La NASA invita a los medios al lanzamiento de Europa Clipper

El X-59 de la NASA avanza en las pruebas de preparación para volar

La NASA invita a creadores de las redes sociales al lanzamiento de la misión Europa Clipper
How to travel at (nearly) the speed of light.
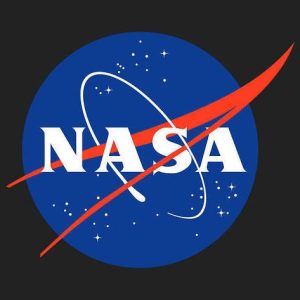
One hundred years ago, on May 29, 1919, measurements of a solar eclipse offered proof for Einstein’s theory of general relativity. Even before that, Einstein had developed the theory of special relativity, which revolutionized the way we understand light. To this day, it provides guidance on understanding how particles move through space — a key area of research to keep spacecraft and astronauts safe from radiation.
The theory of special relativity showed that particles of light, photons, travel through a vacuum at a constant pace of 670,616,629 miles per hour — a speed that’s immensely difficult to achieve and impossible to surpass in that environment. Yet all across space, from black holes to our near-Earth environment, particles are, in fact, being accelerated to incredible speeds, some even reaching 99.9% the speed of light.
Scientists suspect magnetic reconnection is one way that particles are accelerated to nearly light speed. This illustration depicts the magnetic fields around Earth, which snap and realign, causing charged particles to be flung away at high speeds. Find out all three ways that this acceleration happens .
Image Credit: NASA
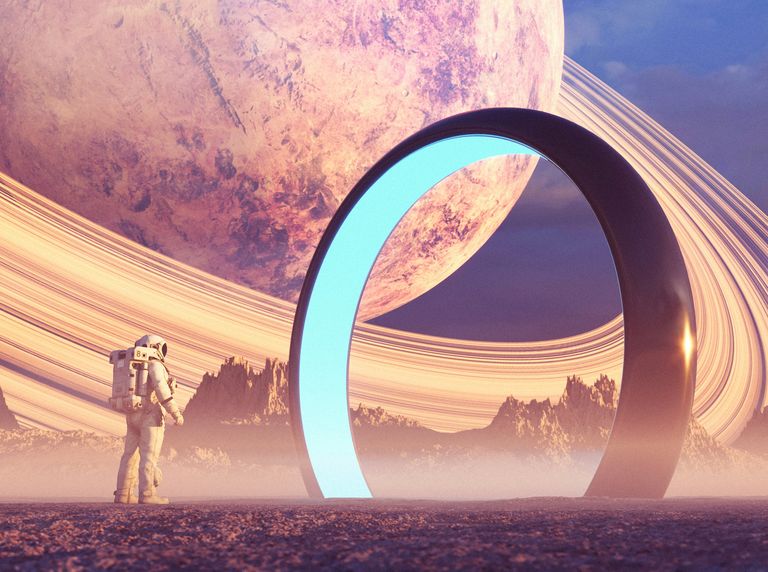
Scientists Believe Light Speed Travel Is Possible. Here’s How.
A functioning warp drive would allow humans to reach the far ends of the cosmos in the blink of an eye.
I n late 2020, physicist Harold “Sonny” White, PhD, research director of the nonprofit Limitless Space Institute , noticed something peculiar—and familiar—in a circular pattern of data plots generated by a recent experiment.
White and his team in LSI’s Houston laboratory were conducting research for the Defense Advanced Research Projects Agency, or DARPA, and had set up these particular experiments to study the energy densities within Casimir cavities, the mysterious spaces between microscopic metal plates in a vacuum. The data plot indicated areas of diminished energy between the plates, which caused them to push toward each other as if trying to fill the void. This is known as negative vacuum energy density, a phenomenon in quantum mechanics called, appropriately enough, the Casimir effect . It’s something that’s helping scientists understand the soupy physics of microscale structures, which some researchers hope can be applied to energy applications that are more practical, such as circuits and electromechanical systems.
But White noticed that the pattern of negative vacuum energy between the plates and around tiny cylindrical columns that they’d inserted in the space looked familiar. It precisely echoed the energy pattern generated by a type of exotic matter that some physicists believe could unlock high-speed interstellar travel. “We then looked, mathematically, at what happens if we placed a one-micron sphere inside of a four-micron cylinder under the same conditions, and found that this kind of structure could generate a little nanoscale warp bubble encapsulating that central region,” White explains.
That’s right—a warp bubble. The essential component of a heretofore fictional warp drive that has for decades been the obsession of physicists, engineers, and sci-fi fans. Warp drive, of course, is the stuff of Star Trek legend, a device enclosed within a spacecraft that gives the mortals aboard the ability to rip around the cosmos at superhuman speed. To the lay sci-fi fan, it’s a “black box”—a convenient, completely made-up workaround to avoid the harsh realities of interstellar travel. However, after decades of speculation, research, and experimentation, scientists believe a warp drive could actually work.
To emphasize: White didn’t actually make a warp bubble. But the data from his study led to an aha moment: For the first time, a buildable warp bubble showed promise of success.
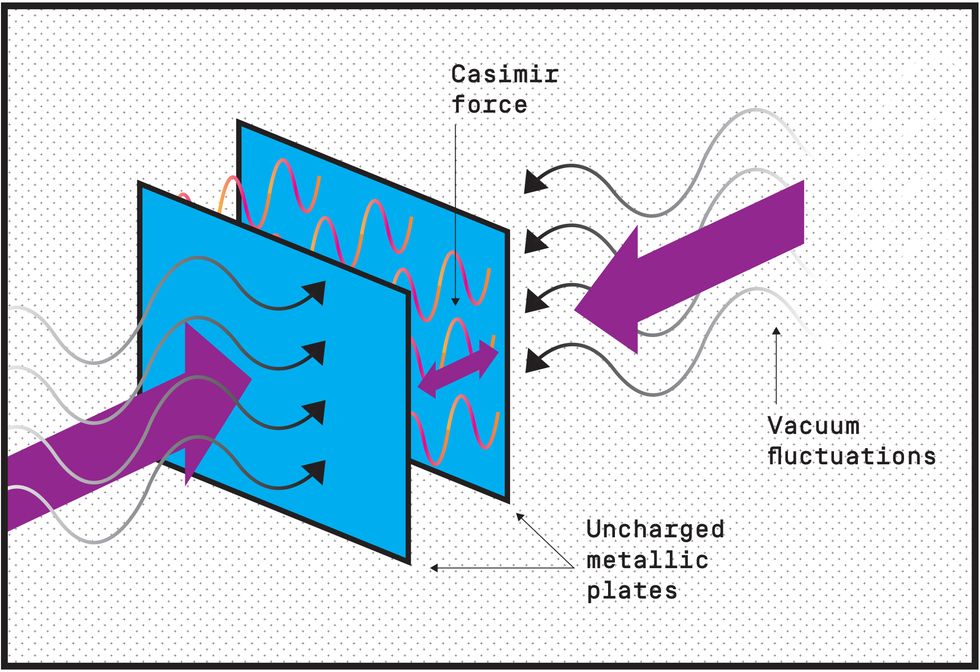
Warp technology’s core science is surprisingly sound. Though the specific mechanics of an actual device haven’t been fully unpacked, the math points toward feasibility. In short, a real-life warp drive would use massive amounts of energy, which can come in the form of mass, to create enough gravitational pull to distort spacetime in a controlled fashion, allowing a ship to speed along inside a self-generated bubble that itself is able to travel at essentially any speed. Warp drives popped up in fiction intermittently for several decades before Star Trek creator Gene Roddenberry plugged one into the USS Enterprise in 1966. But Miguel Alcubierre, PhD, a Mexican theoretical physicist and professed Star Trek enthusiast, gave the idea real-world legs when he released a paper in 1994 speculating that such a drive was mathematically possible. It was the first serious treatment of a warp drive’s feasibility, and it made headlines around the world. His breakthrough inspired more scientists to nudge the theoretical aspects of warp drive toward concrete, practical applications.
“I proposed a ‘geometry’ for space that would allow faster-than-light travel as seen from far away, essentially expanding space behind the object we want to move and contracting it in front,” Alcubierre says. “This forms a ‘bubble’ of distorted space, inside of which an object—a spaceship, say—could reside.”
Physicists tend to speak in relative terms. By injecting the sly qualifier “as seen from far away,” Alcubierre might sound like he’s describing the galactic equivalent of an optical illusion —an effect perhaps similar to driving past a truck going the opposite direction on the highway when you’re both going 60 miles an hour. Sure feels like a buck-twenty, doesn’t it? But the A-to-B speed is real; the warp effect simply shortens the literal distance between two points. You’re not, strictly speaking, moving faster than light. Inside the bubble, all appears relatively normal, and light moves faster than you are, as it should. Outside the bubble, however, you’re haulin’ the mail.
THOUGH THE SPECIFIC MECHANICS OF AN ACTUAL DEVICE HAVEN’T BEEN UNPACKED, THE MATH POINTS TOWARD FEASIBILITY.
Alcubierre’s proposal had solved one of the initial hurdles to achieving warp speeds: The very idea clashes with Einstein’s long-accepted theory of general relativity, which states that nothing can travel faster than the speed of light, but it doesn’t preclude space itself from traveling faster than that. In fact, scientists speculate that the same principles explain the rapid expansion of the universe after the Big Bang .
While concluding that warp speed was indeed possible, Alcubierre also found that it would require an enormous amount of energy to sustain the warp bubble. He theorized that negative energy—the stuff hinted at by White’s experimentation with Casimir cavities—could be a solution. The only problem is that no one has yet proved that negative energy is real. It’s the unobtanium of our spacefaring imaginations, something researchers only believe to exist. In theory, however, this unknown matter may be sufficiently powerful that future warp drive designers could channel it to contract spacetime around it. In conceptual drawings of warp-capable spacecraft , enormous material rings containing this energy source surround a central fuselage. When activated, it warps spacetime around the entire ship. The more intense the warping, the faster the warp travel is achieved.
Of course, it’s not that simple. Physicist José Natário, PhD, a professor at the Instituto Superior Técnico in Lisbon, wrote his own influential paper about the mathematical feasibility of warp drives in 2001. However, he is concerned about practical conundrums, like the amount of energy required. “You need to be able to curve spacetime quite a lot in order to do this,” he says. “We’re talking about something that would be much, much more powerful than the sun.”
Alcubierre is similarly skeptical that his theoretical ideas might ever be used to develop a working warp drive. “In order to have a bubble about 100 meters wide traveling at precisely the speed of light, you would need about 100 times the mass of the planet Jupiter converted into negative energy, which of course sounds absurd,” he says. By that standard, he concludes, a warp drive is very unlikely.
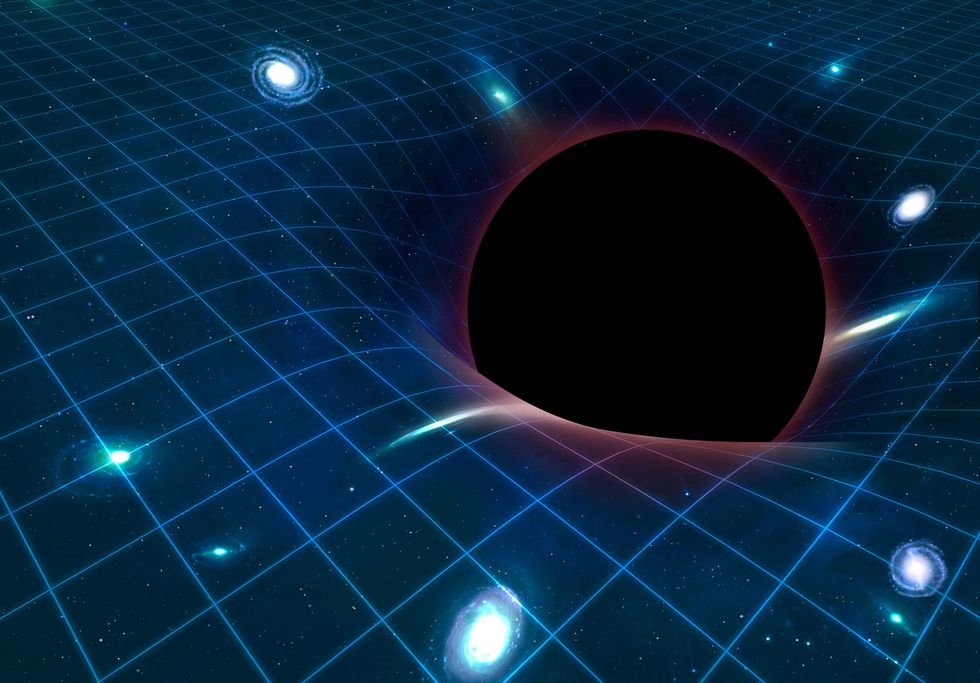
Physicists love a challenge, though. In the 29 years since Alcubierre published his paper, other scientists have wrestled with the implications of the work, providing alternative approaches to generating the energy using more accessible power sources, finding oblique entry points to the problem, and batting ideas back and forth in response to one another’s papers. They use analogies involving trampolines , tablecloths, bowling balls, balloons, conveyor belts, and music to explain the physics.
They even have their own vocabulary. It’s not faster-than-light travel; it’s superluminal travel, thank you. Then there’s nonphysical and physical—a.k.a. the critical distinction between theoretical speculation and something that can actually be engineered. (Pro tip: We’re aiming for physical here, folks.) They do mention Star Trek a lot, but never Star Wars . Even the scruffiest-looking nerf herder knows that the ships in Star Wars use hyperdrive, which consumes fuel, rather than warp drives, which don’t use propulsive technology but instead rely on, well, warping. They’re also vague about details like what passengers would experience, what gravity is like on board since you’re carrying around boatloads of energy, and what would happen if someone, say, jumped out of the ship while warping. (A speculative guess: Nothing good.)
Such research isn’t typically funded by academic institutions or the DARPAs and NASAs of the world, so much of this work occurs in the scientists’ spare time. One such scientist and Star Trek enthusiast is physicist Erik Lentz, PhD. Now a researcher at Pacific Northwest National Laboratory in Richland, Washington, Lentz was doing postdoctoral work at Göttingen University in Germany when, amid the early, isolated days of the pandemic, he mulled the idea of faster-than-light travel. He published a paper in 2021 arguing that warp drives could be generated using positive energy sources instead of the negative energy that Alcubierre’s warp drive seemed to require.
“There are a number of barriers to entry to actually being able to build a warp drive,” Lentz says. “The negative energy was the most obvious, so I tried to break that barrier down.”
He explored a new class of solutions in Einstein’s general relativity while focusing on something called the weak-energy condition, which, he explains, tracks the positivity of energy in spacetime. He hit upon a “soliton solution”—a wave that maintains its shape and moves at a constant velocity—that could both satisfy the energy-level challenge and travel faster than light. Such a warp bubble could travel along using known energy sources, though harnessing those at the levels needed are still far beyond our capabilities. The next step, he notes, may be bringing the energy requirements for a warp drive to within the range of a nuclear fusion reactor.
A fusion-powered device could theoretically travel to and from Proxima Centauri , Earth’s nearest star, in years instead of decades or millennia, and then go faster and faster as power sources improve. Current conventional rocket technology, on the other hand, would take 50,000 years just for a one-way trip—assuming, of course, there was an unlimited fuel supply for those engines.
“IF YOU COLLIDE WITH SOMETHING ON YOUR PATH, IT WOULD ALMOST CERTAINLY BE CATASTROPHIC.”
Like Alcubierre’s original thesis, Lentz’s paper had a seismic impact on the warp drive community, prompting yet another group of scientists to dig into the challenge. Physicist Alexey Bobrick and technology entrepreneur Gianni Martire have been particularly prolific. In 2021, they released a paper theorizing that a class of subliminal warp drives, traveling at just a fraction of light speed, could be developed from current scientific understanding. While that paper essentially argued that it’s perfectly acceptable to walk before you can run, they followed it up with another theory earlier this year that describes how a simulated black hole , created using sound waves and glycerin and tested with a laser beam, could be used to evaluate the levels of gravitational force needed to warp spacetime. The duo coded that breakthrough into a public app that they hope will help more quickly push theoretical ideas to practical ones. Though the team is waiting for the technology to clear a peer review stage before releasing details, the app is essentially a simulator that allows scientists to enter their warp-speed equations to validate whether they’re practical.
“When somebody publishes a warp metric for the first time, people say, ‘Okay, is your metric physical?’” Martire says. The answer to that question—whether the metric has practical potential or is strictly theoretical—is hard to establish given the challenges of testing these hypotheses. That determination could take six to eight months. “Now we can tell you within seconds, and it shows you visually how off you are or how close you are,” he says.
While useful, the app will speed up the preliminary math only for future researchers. Galaxy-sized challenges remain before we ever experience turbocharged interstellar travel. Alcubierre worries in particular about what may happen near the walls of the warp bubble. The distortion of space is so violent there, he notes, that it would destroy anything that gets close. “If you collide with something on your path, it would almost certainly be catastrophic,” he says.
Natário mulls even more practical issues, like steering and stopping. “It’s a bubble of space, that you’re pushing through space,” he says. “So, you’d have to tell space ... to curve in front of your spaceship.” But therein lies the problem: You can’t signal to the space in front of you to behave the way you want it to.
His opinion? Superluminal travel is impossible. “You need these huge deformations that we have no idea how to accomplish,” Natário says. “So yes, there has been a lot of effort toward this and studying these weird solutions, but this is all still completely theoretical, abstract, and very, very, very, very far from getting anywhere near a practical warp drive.” That’s “very” to the power of four, mind you—each crushing blow pushing us exponentially, excruciatingly further and further away from our yearned-for superluminal lives.
Ultimately, the pursuit of viable high-speed interstellar transportation also points to a more pressing terrestrial challenge: how the scientific community tackles ultra-long-term challenges in the first place. Most of the research so far has come from self-starters without direct funding, or by serendipitous discoveries made while exploring often unrelated research, such as Dr. White’s work on Casimir cavities.
Many scientists argue that we’re in a multi-decade period of stagnation in physics research, and warp drive—despite its epic time horizons before initial research leads to galaxy-spanning adventures—is somewhat emblematic of that stagnation. Sabine Hossenfelder, a research fellow at the Frankfurt Institute for Advanced Studies and creator of the YouTube channel Science Without the Gobbledygook , noted in a 2020 blog post that physics research has drifted away from frequent, persistent physical experimentation to exorbitant infusions of cash into relatively few devices. She writes that with fewer experiments, serendipitous discoveries become increasingly unlikely. Without those discoveries, the technological progress needed to keep experiments economically viable never materializes.
When asked whether this applied equally to warp drive, Hossenfelder sees a faint but plausible connection. “Warp drives are an idea that is not going to lead to applications in the next 1,000 years or so,” she says. “So they don’t play a big role in that one way to another. But when it comes to the funding, you see some overlap in the problems.”
So, despite all the advances, the horizon for a warp drive remains achingly remote. That hasn’t fazed the scientists involved, though. A few years ago, while teaching in France, White visited the Strasbourg Cathedral with his wife. While admiring its 466-foot-tall spire, he was struck by the fact that construction began in 1015 but didn’t wrap up until 1439—a span of 424 years. Those who built the basement had no chance of ever seeing the finished product, but they knew they had to do their part to aid future generations. “I don’t have a crystal ball,” White says. “I don’t know what the future holds. But I know what I need to be doing right now.”
Eric Adams is a writer and photographer who focuses on technology, transportation, science, travel, and other subjects for a wide range of outlets, including Wired, The Drive, Gear Patrol, Men's Health, Popular Science, Forbes, and others.
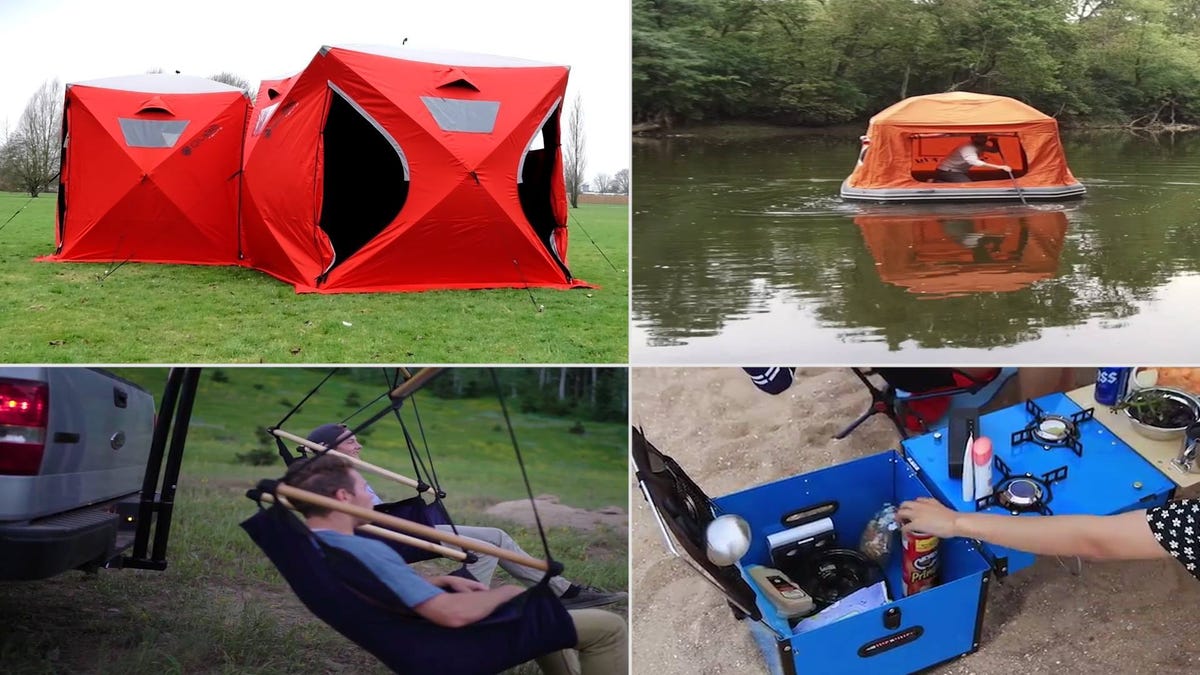
Pop Mech Pro
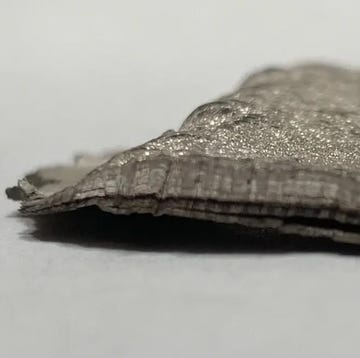
Could This Metal Shard Be Alien Technology?
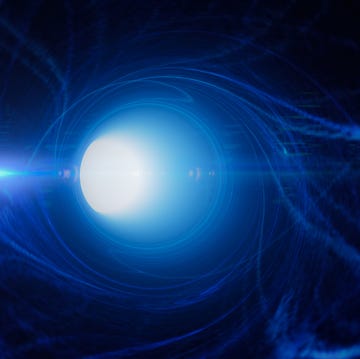
Wormholes May Be a Portal for Interstellar Travel
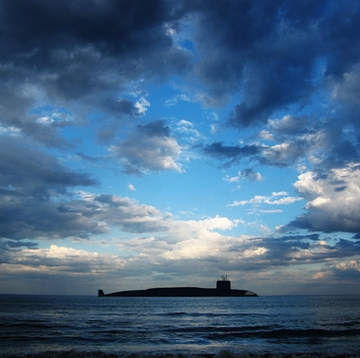
What We Know About India’s Secret New Submarine
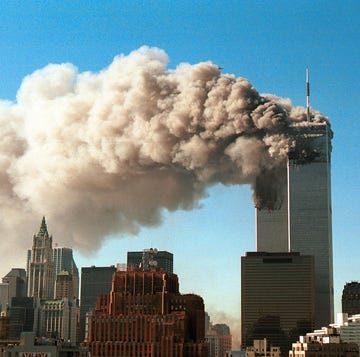
Debunking the 9/11 Myths: The Airplanes
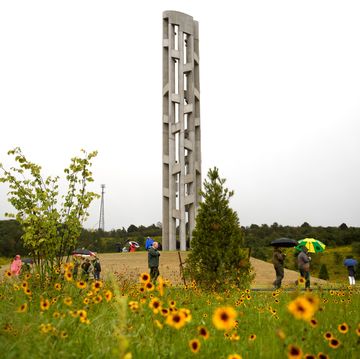
We Debunked the 9/11 Myths About United Flight 93
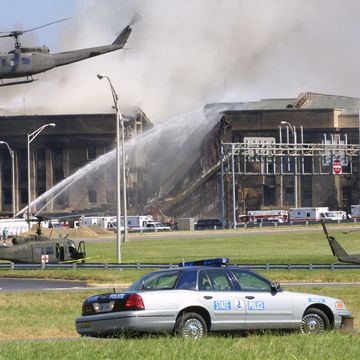
Myths About the 9/11 Pentagon Attack: Debunked
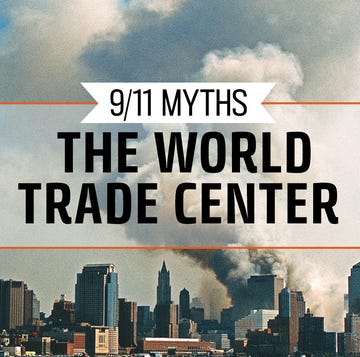
Debunking the 9/11 Myths: The World Trade Center
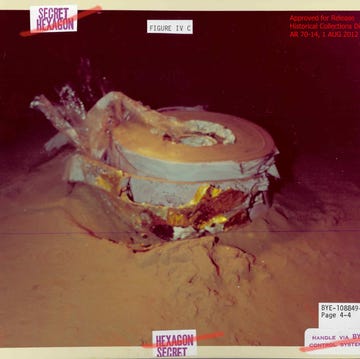
America’s Secret Race to Recover a Spy Satellite
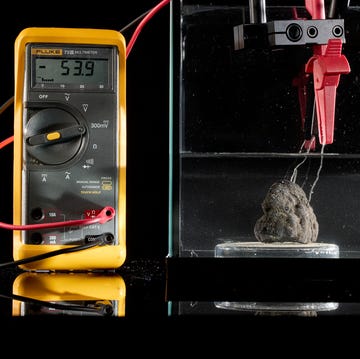
Scientists Find Groundbreaking Deep-Sea Battery
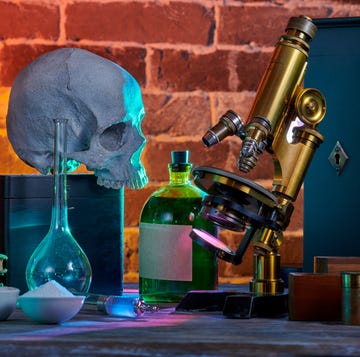
Archaeologists Find an Ancient Immortality Potion
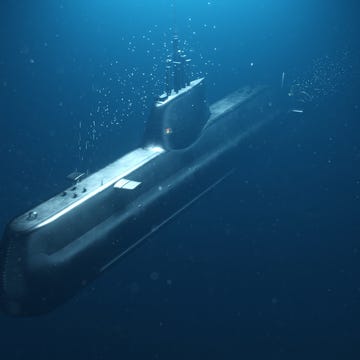
China Could Build the World’s Fastest Submarines
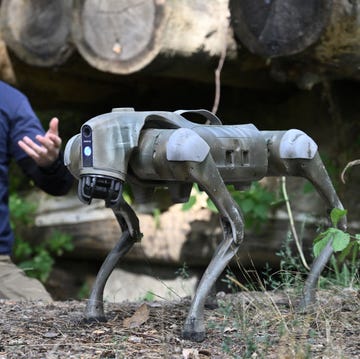
Robot Dogs Could Make Appearance in Ukraine-Russia
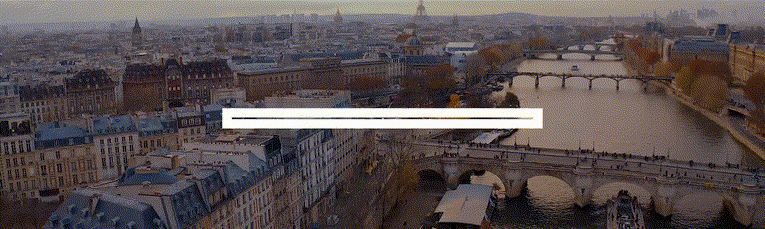
IMAGES
VIDEO
COMMENTS
The speed of light in vacuum, commonly denoted c, is a universal physical constant that is exactly equal to 299,792,458 metres per second (approximately 300,000 kilometres per second; 186,000 miles per second; 671 million miles per hour). [Note 3] According to the special theory of relativity, c is the upper limit for the speed at which conventional matter or energy (and thus any signal ...
In fact, we now define the speed of light to be a constant, with a precise speed of 299,792,458 meters per second. While it remains a remote possibility in deeply theoretical physics that light ...
The theory of special relativity showed that particles of light, photons, travel through a vacuum at a constant pace of 670,616,629 miles per hour — a speed that's immensely difficult to achieve and impossible to surpass in that environment. ... A well-aimed near-light-speed particle can trip onboard electronics and too many at once could ...
The speed of light in a vacuum is 186,282 miles per second (299,792 kilometers per second), and in theory nothing can travel faster than light.
The final step is to calculate the total distance that the light has traveled within the time. You can calculate this answer using the speed of light formula: distance = speed of light × time. Thus, the distance that the light can travel in 100 seconds is 299,792,458 m/s × 100 seconds = 29,979,245,800 m. FAQs.
According to Albert Einstein 's theory of special relativity, summarized by the famous equation E = mc2, the speed of light (c) is something like a cosmic speed limit that cannot be surpassed. So, light-speed travel and faster-than-light travel are physical impossibilities, especially for anything with mass, such as spacecraft and humans.
That's because all massless particles are able to travel at this speed, and since light is massless, it can travel at that speed. And so, the speed of light became an important cornerstone of ...
So, what is the speed of light? Light moves at an incredible 186,000 miles per second (300,000 kilometers per second), equivalent to almost 700 million mph (more than 1 billion km/h). That's fast ...
Speed of Light (c): Light travels at a constant speed of 1,079,252,848.8 (1.07 billion) km per hour. That works out to 299,792,458 m/s, or about 670,616,629 mph (miles per hour). To put that in ...
Speed of light, speed at which light waves propagate through different materials. In a vacuum, the speed of light is 299,792,458 meters per second. ... Ask the Chatbot Games & Quizzes History & Society Science & Tech Biographies Animals & Nature Geography & Travel Arts & Culture Money Videos. speed of light. Table of Contents
The speed of light is sometimes referred to as the "cosmic speed limit". Light travels in a vacuum at 186,282.4 miles per second or 299,792,458 meters/second. For simplicity, it is often said ...
The speed of light is the rate at which light travels. The speed of light in a vacuum is a constant value that is denoted by the letter c and is defined as exactly 299,792,458 meters per second. Visible light, other electromagnetic radiation, gravity waves, and other massless particles travel at c. Matter, which has mass, can approach the speed ...
We know that light has a finite speed and it travels at the speed of 300,000 kilometers per second. This a great distance to travel. On earth this speed is almost instantaneous.
It is often stated that the speed of light is constant and that nothing can travel faster than the speed of light. This isn't entirely accurate. The value of 299,792,458 meters per second (186,282 miles per second) is the speed of light in a vacuum. However, light actually slows down as it passes through different media.
Today the speed of light, or c as it's commonly known, is considered the cornerstone of special relativity - unlike space and time, the speed of light is constant, independent of the observer. What's more, this constant underpins much of what we understand about the Universe. It matches the speed of a gravitational wave, and yes, it's the ...
The speed of light is the Universal speed limit - nothing can travel faster than light.In the vacuum (commonly denoted c), its exact value is 299,792,458 meters per second (around 186,000 miles per second). In other words, if you could travel at the speed of light, you could go around the Earth 7.5 times in one second.
The speed of light, which is almost 300,000 km per second, can be manipulated by changing media or with quantum interference. ... (see Figure 2), although the frequency remains unaltered. Light travels at approximately 300,000 kilometers per second in a vacuum, which has a refractive index of 1.0, but it slows down to 225,000 kilometers per ...
The speed of light in vacuum is constant and does not depend on characteristics of the wave (e.g. its frequency, polarization, etc). In other words, in vacuum blue and red colored light travel at the same speed c.. The propagation of light in a medium involves complex interactions between the wave and the material through which it travels.
Light travels at a constant, finite speed of 186,000 mi/sec. A traveler, moving at the speed of light, would circum-navigate the equator approximately 7.5 times in one second. By comparison, a traveler in a jet aircraft, moving at a ground speed of 500 mph, would cross the continental U.S. once in 4 hours. ...
Light Speed. Light is fast! It can reach the universal speed limit — 186,000 miles per second. (If you could travel as fast as light, the universe would look very different.) Because it moves so quickly, light can seem to appear instantaneously. Think about when you turn on a TV... images pop up right away.
The theory of special relativity showed that particles of light, photons, travel through a vacuum at a constant pace of 670,616,629 miles per hour — a speed that's immensely difficult to achieve and impossible to surpass in that environment. Yet all across space, from black holes to our near-Earth environment, particles are, in fact, being ...
We can slow it down by focusing it through prisims or crystals. But it still is traveling at 186,000/MPS.and that speed does not change. So, that is why we can see the outer edge of the universe ...
Here's How. Scientists Believe Light Speed Travel Is Possible. Here's How. A functioning warp drive would allow humans to reach the far ends of the cosmos in the blink of an eye. I n late 2020 ...